Autosomal Dominant Polycystic Kidney Disease via the PKD1 Gene
Summary and Pricing 
Test Method
Exome Sequencing with CNV DetectionTest Code | Test Copy Genes | Test CPT Code | Gene CPT Codes Copy CPT Code | Base Price | |
---|---|---|---|---|---|
10453 | PKD1 | 81407 | 81407,81479 | $990 | Order Options and Pricing |
Pricing Comments
Our favored testing approach is exome based NextGen sequencing with CNV analysis. This will allow cost effective reflexing to PGxome or other exome based tests. However, if full gene Sanger sequencing is desired for insurance or other reasons, please see link below for Test Code, pricing, and turnaround time information. If the Sanger option is selected, CNV detection may be ordered through Test #2058.
An additional 25% charge will be applied to STAT orders. STAT orders are prioritized throughout the testing process.
Click here for costs to reflex to whole PGxome (if original test is on PGxome Sequencing platform).
Click here for costs to reflex to whole PGnome (if original test is on PGnome Sequencing platform).
The Sanger Sequencing method for this test is NY State approved.
For Sanger Sequencing click here.Turnaround Time
3 weeks on average for standard orders or 2 weeks on average for STAT orders.
Please note: Once the testing process begins, an Estimated Report Date (ERD) range will be displayed in the portal. This is the most accurate prediction of when your report will be complete and may differ from the average TAT published on our website. About 85% of our tests will be reported within or before the ERD range. We will notify you of significant delays or holds which will impact the ERD. Learn more about turnaround times here.
Targeted Testing
For ordering sequencing of targeted known variants, go to our Targeted Variants page.
Clinical Features and Genetics 
Clinical Features
Autosomal dominant polycystic kidney disease (ADPKD) is a common inherited kidney disease with multisystem involvement. ADPKD is characterized by bilateral renal cysts accompanied by cysts in other organs including the liver, seminal vesicles, pancreas, and arachnoid membrane (Harris et al. 2011. PubMed ID: 20301424). Renal symptoms include hypertension, renal pain, and renal insufficiency. Nearly half of ADPKD patients have end-stage renal disease (ESRD) by age 60 years. The progressive growth of liver cysts is the most common extrarenal manifestation of ADPKD. The most important non-cystic manifestations of ADPKD are vascular and cardiac abnormalities including intracranial aneurysms, mitral valve prolapse, dilatation of the aortic root, dissection of the thoracic aorta, and abdominal wall hernias. The clinical spectrum of ADPKD is wide and substantial variability of disease severity can occur even within the same family.
Patients with ADPKD typically have onset of symptoms in adulthood. In some rare cases, however, patients with bi-allelic PKD1 variants may have clinical features similar to those of patients with autosomal recessive polycystic kidney disease (ARPKD) (Rossetti et al. 2009. PubMed ID: 19165178; Vujic et al. 2010. PubMed ID: 20558538; Audrézet et al. 2016. PubMed ID: 26139440). In these rare cases, symptoms may appear in early childhood or even in utero.
Genetics
PKD1 and PKD2 are the two major causative genes for ADPKD (Rossetti et al. 2007. PubMed ID: 17582161; Audrézet et al. 2012. PubMed ID: 22508176). Accounting for a small fraction of ADPKD cases, GANAB and DNAJB11 are newly implicated in ADPKD (Porath et al. 2016. PubMed ID: 27259053; Cornec-Le Gall et al. 2018. PubMed ID: 29706351).
PKD1 (46 coding exons) encodes a member of the polycystin protein family, which plays an important role in renal tubular development. Genetic defects in PKD1 account for approximately 85% of genetically positive ADPKD cases and have been found across the whole coding region of the gene (Rossetti et al. 2007. PubMed ID: 17582161; Audrézet et al. 2012. PubMed ID: 22508176). In addition to missense substitutions and small in-frame changes, truncating changes (nonsense, canonical splice variants and frame-shifting small deletion/insertions) are the majority of PKD1 defects. Gross deletions have been also reported, but are relatively rare (<4% of pathogenic variants) (Ariyurek et al. 2004. PubMed ID: 14695542; Rossetti et al. 2007. PubMed ID: 17582161; Audrézet et al. 2012. PubMed ID: 22508176). Our internal data suggested gene conversions are rare (<0.5%) in PKD1.
The majority of PKD1 defects were found in single patients (Audrézet et al. 2012. PubMed ID: 22508176). De novo pathogenic variants account for about 10% of individuals with ADPKD in adulthood (Neumann et al. 2012. PubMed ID: 22367170).
Clinical Sensitivity - Sequencing with CNV PGxome
In two large cohort studies, the overall pathogenic variant detection rate of PKD1 and PKD2 is about 89%, in which defects in PKD1 and PKD2 explain approximately 85% and 15% of genetically positive autosomal dominant polycystic kidney disease (ADPKD) cases, respectively (Rossetti et al. 2007. PubMed ID: 17582161; Audrézet et al. 2012. PubMed ID: 22508176). Large deletions and duplications in PKD1 and PKD2 are relatively rare (<4%) in ADPKD patients (Bataille et al. 2011. PubMed ID: 22008521; Audrézet et al. 2012. PubMed ID: 22508176).
Our sequencing method is expected to be nearly equal to the reported overall detection rate of PKD1 pathogenic variants in ADPKD due to the rarity of gross deletions in this gene. Copy number variants (CNVs) analysis for the homologous region (exons 1 to 33) of PKD1 via the multiplex ligation-dependent amplification (MLPA) assay, which can be ordered separately (Test #2058), can increase the detection rate of PKD1 pathogenic variants.
Since we primarily use Next Generation Sequencing (NGS) to test the PKD1 gene (see Testing Strategy section), gene conversions can be missed. However, our internal data suggested gene conversions are rare (<0.5%) in PKD1. These events have been found by long-range PCR based Sanger sequencing, but not by NGS only. Therefore, to increase detection rate (but by a very limited amount) of PKD1 pathogenic variants, Sanger sequencing for exons 1 to 33 (homologous regions) of PKD1 may be ordered.
Testing Strategy
This test is performed using Next-Gen sequencing with additional Sanger sequencing as necessary.
DNA analysis of the PKD1 gene is complicated and challenging due to the presence of several PKD1 pseudogenes. There is high sequence similarity of exons 1 to 33 between PKD1 and its pseudogenes (Audrézet et al. 2012. PubMed ID: 22508176). We have validated Next Generation Sequencing (NGS) to reliably sequence these exons.
For the PKD1 gene, including exons 1 to 33 (homologous regions), we primarily use Next Generation Sequencing (NGS) (~96%) complimented with Sanger sequencing for low-coverage regions (~4%). For any pathogenic, likely pathogenic, and uncertain variants found in exons 1 to 33 (homologous regions) via NGS, we use long-range PCR based Sanger sequencing to confirm them. Therefore, this test provides full coverage of all coding exons of the PKD1 gene plus 10 bases of flanking noncoding DNA in all available transcripts along with other non-coding regions in which pathogenic variants have been identified at PreventionGenetics or reported elsewhere. We define full coverage as >20X NGS reads or Sanger sequencing. PGnome panels typically provide slightly increased coverage over the PGxome equivalent. PGnome sequencing panels have the added benefit of additional analysis and reporting of deep intronic regions (where applicable).
Due to homologous sequence, gene conversion events in the PKD1 gene have been reported in the literature and found at PreventionGenetics. Our internal data suggested gene conversions are rare (<0.5%) in PKD1. These events have been found by long-range PCR based Sanger sequencing, but not by NGS only. Therefore, Sanger sequencing for exons 1 to 33 (homologous regions) of PKD1 may also be ordered.
Regarding copy number variants (CNVs) analysis, because of the paucity of CNVs and the complicated nature of sequence in PKD1, CNV analysis for this gene can be performed via the multiplex ligation-dependent amplification (MLPA) assay with limited increased sensitivity (compared to Next-Gen sequencing CNV analysis), and can be ordered separately (Test #2058).
Dependent on the sequencing backbone selected for this testing, discounted reflex testing to any other similar backbone-based test is available (i.e., PGxome panel to whole PGxome; PGnome panel to whole PGnome).
Indications for Test
Candidates for this test are patients with ADPKD. Targeted Sanger testing is indicated for family members of patients who have known PKD1 pathogenic variants.
Candidates for this test are patients with ADPKD. Targeted Sanger testing is indicated for family members of patients who have known PKD1 pathogenic variants.
Gene
Official Gene Symbol | OMIM ID |
---|---|
PKD1 | 601313 |
Inheritance | Abbreviation |
---|---|
Autosomal Dominant | AD |
Autosomal Recessive | AR |
X-Linked | XL |
Mitochondrial | MT |
Disease
Name | Inheritance | OMIM ID |
---|---|---|
Polycystic Kidney Disease 1 | AD | 173900 |
Citations 
- Ariyurek et al. 2004. PubMed ID: 14695542
- Audrézet et al. 2012. PubMed ID: 22508176
- Audrézet et al. 2016. PubMed ID: 26139440
- Bataille et al. 2011. PubMed ID: 22008521
- Cornec-Le Gall et al. 2018. PubMed ID: 29706351
- Harris et al. 2011. PubMed ID: 20301424
- Neumann et al. 2012. PubMed ID: 22367170
- Porath et al. 2016. PubMed ID: 27259053
- Rossetti et al. 2007. PubMed ID: 17582161
- Rossetti et al. 2009. PubMed ID: 19165178
- Vujic et al. 2010. PubMed ID: 20558538
Ordering/Specimens 
Ordering Options
We offer several options when ordering sequencing tests. For more information on these options, see our Ordering Instructions page. To view available options, click on the Order Options button within the test description.
myPrevent - Online Ordering
- The test can be added to your online orders in the Summary and Pricing section.
- Once the test has been added log in to myPrevent to fill out an online requisition form.
- PGnome sequencing panels can be ordered via the myPrevent portal only at this time.
Requisition Form
- A completed requisition form must accompany all specimens.
- Billing information along with specimen and shipping instructions are within the requisition form.
- All testing must be ordered by a qualified healthcare provider.
For Requisition Forms, visit our Forms page
If ordering a Duo or Trio test, the proband and all comparator samples are required to initiate testing. If we do not receive all required samples for the test ordered within 21 days, we will convert the order to the most effective testing strategy with the samples available. Prior authorization and/or billing in place may be impacted by a change in test code.
Specimen Types
Specimen Requirements and Shipping Details
PGxome (Exome) Sequencing Panel
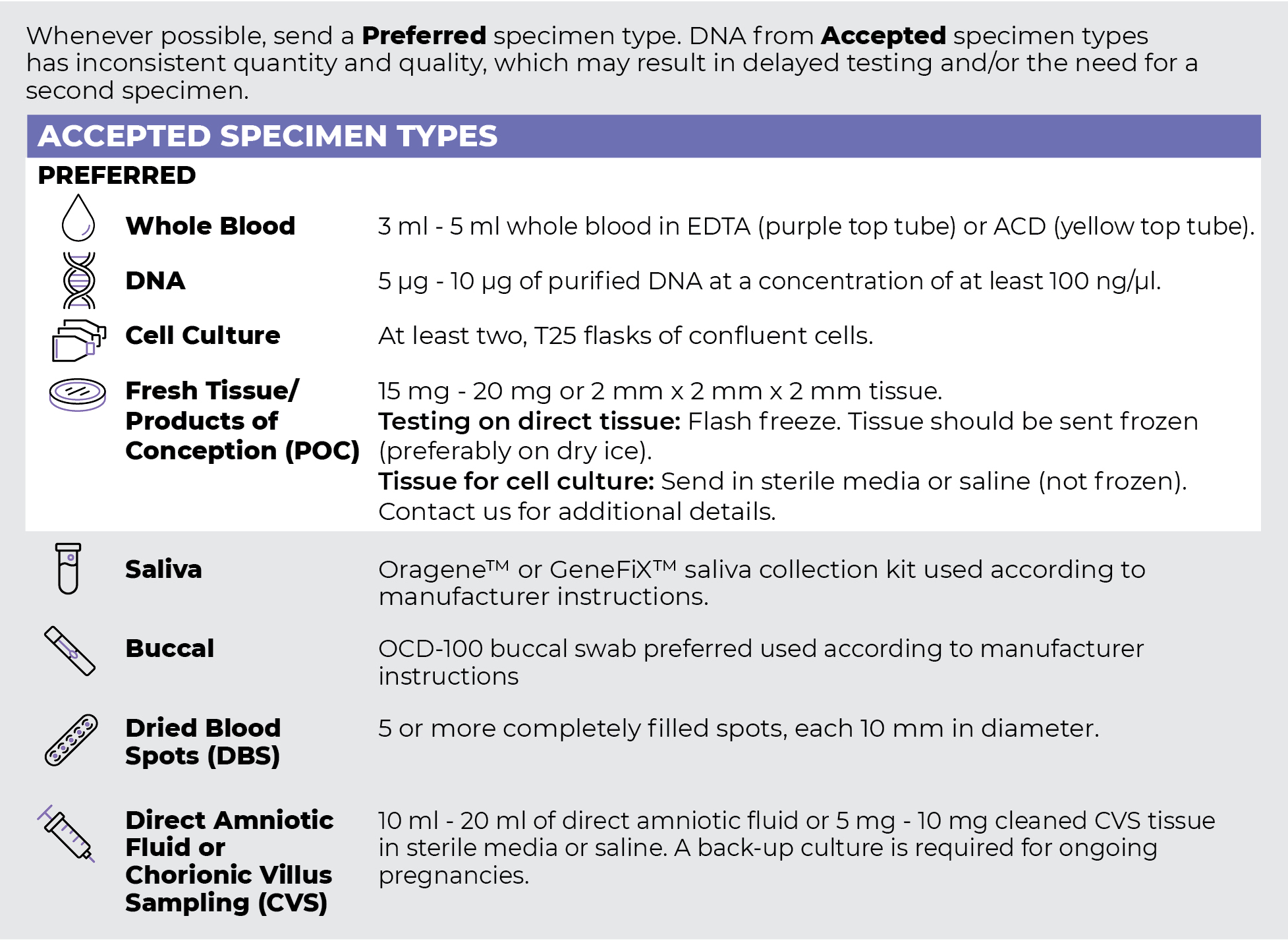
PGnome (Genome) Sequencing Panel
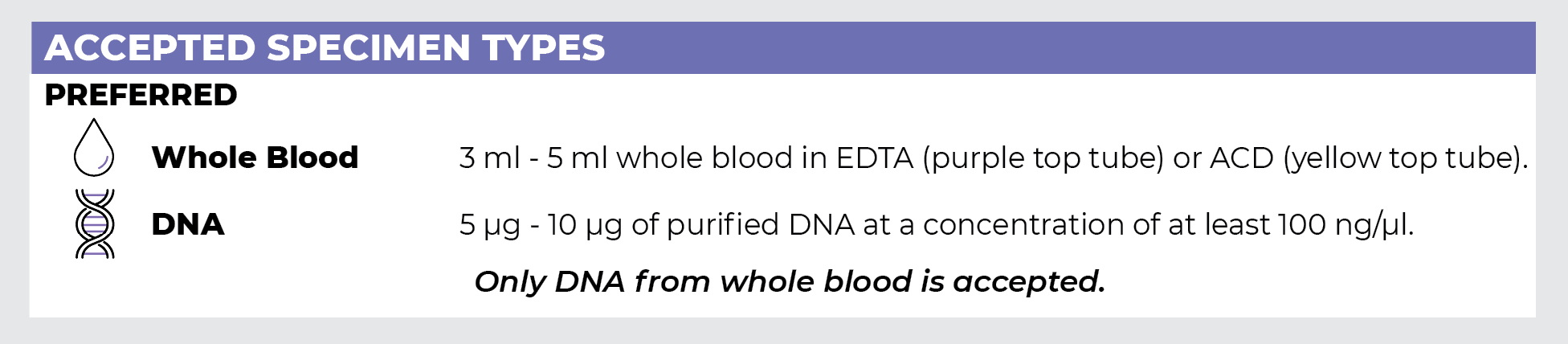
ORDER OPTIONS
View Ordering Instructions1) Select Test Type
2) Select Additional Test Options
No Additional Test Options are available for this test.