Severe Congenital Neutropenia and Neutrophilia via the CSF3R Gene
Summary and Pricing 
Test Method
Exome Sequencing with CNV DetectionTest Code | Test Copy Genes | Test CPT Code | Gene CPT Codes Copy CPT Code | Base Price | |
---|---|---|---|---|---|
8135 | CSF3R | 81479 | 81479,81479 | $990 | Order Options and Pricing |
Pricing Comments
Our favored testing approach is exome based NextGen sequencing with CNV analysis. This will allow cost effective reflexing to PGxome or other exome based tests. However, if full gene Sanger sequencing is desired for STAT turnaround time, insurance, or other reasons, please see link below for Test Code, pricing, and turnaround time information. If the Sanger option is selected, CNV detection may be ordered through Test #600.
An additional 25% charge will be applied to STAT orders. STAT orders are prioritized throughout the testing process.
Click here for costs to reflex to whole PGxome (if original test is on PGxome Sequencing platform).
Click here for costs to reflex to whole PGnome (if original test is on PGnome Sequencing platform).
The Sanger Sequencing method for this test is NY State approved.
For Sanger Sequencing click here.Turnaround Time
3 weeks on average for standard orders or 2 weeks on average for STAT orders.
Please note: Once the testing process begins, an Estimated Report Date (ERD) range will be displayed in the portal. This is the most accurate prediction of when your report will be complete and may differ from the average TAT published on our website. About 85% of our tests will be reported within or before the ERD range. We will notify you of significant delays or holds which will impact the ERD. Learn more about turnaround times here.
Targeted Testing
For ordering sequencing of targeted known variants, go to our Targeted Variants page.
Clinical Features and Genetics 
Clinical Features
Severe Congenital Neutropenia (SCN) is a disorder of neutrophil production with varying symptoms and modes of inheritance. SCN is characterized by absolute neutrophil counts (ANC) consistently below 500/microL and severe systemic bacterial infections beginning in early infancy (Boxer and Newburger 2007). Other symptoms include recurrent fevers, sinusitis, gingivitis and other soft tissue infections. A hallmark of SCN is bone marrow maturation arrest; neutrophils differentiate only to the promyelocyte/myelocyte stage (Kostman 1975). About 95% of patients respond to treatment with recombinant granulocyte-colony stimulating factor (G-CSF) with an increase in ANC (Bellanne-Chantelot et al. 2004; Freedman et al. 2000). SCN is a premalignant condition, and patients are at an elevated risk of developing myelodysplastic syndrome (MDS) and acute myeloblastic leukemia (AML). The risk of developing a malignancy increases upon G-CSF treatment (Gilman et al. 1970; Freedman et al. 2000; Rosenberg et al. 2006). The cumulative incidence of leukemia in SCN patients is ~ 22% after 15 years of G-CSF treatment (Rosenberg et al. 2010). In contrast to neutropenia, neutrophilia is characterized by an increase in neutrophils and is generally defined as an absolute neutrophil count greater than 7700/microL.
Genetics
Causative variants in the ELANE gene, consisting primarily of missense variants, are associated with 35%-63% of SCN cases (Rosenberg et al. 2006; Bellanne-Chantelot et al. 2004) and are inherited in an autosomal dominant manner. Pathogenic variants in the GFI1 gene have also been identified in a small fraction of patients with autosomal dominant SCN (Person et al. 2003). GATA2 gene variants are also inherited in an autosomal dominant manner and are associated with neutropenia, MonoMac syndrome, and frequent evolution to MDS/AML (Pasquet et al. 2013). Autosomal recessive forms of SCN have been linked to variants in the HAX1 (Klein et al. 2007), G6PC3 (Boztug et al. 2009), CSF3R (Dong et al. 1995; Triot et al. 2014), and VPS45 (Stepensky et al. 2013) genes, and X-linked SCN has been attributed to variants in the WAS gene (Ancliff et al. 2006). In one recent study, a genetic basis of SCN was not identified in approximately 40% of the cases analyzed (Xia et al. 2009). These data suggest that additional genetic causes of SCN have yet to be discovered and that testing for other disorders with neutropenia as a common symptom, e.g. Hermansky-Pudlak syndrome type 2 (HPS2) and Chediak-Higashi syndrome (CHS), should also be considered. The mechanism of neutropenia is due, at least in some cases, to increased apoptosis of myeloid cells. Gene defects in ELANE and G6PC3 are associated with increased endoplasmic reticulum stress and the unfolded protein response (Xia and Link 2008; Klein 2011), whereas deficiencies of the mitochondrial protein HAX1 cause apoptosis through disruption of mitochondrial membrane potential (Klein 2011).
In contrast to other forms of SCN, inherited biallelic missense / nonsense variants, and small deletions in CSF3R were shown recently to be direct causes of SCN in patients who had full myeloid cell maturation in bone marrow, but displayed peripheral neutropenia (Triot et al. 2014). The CSF3R gene encodes the cell-surface granulocyte colony stimulating factor (G-CSF) receptor (G-CSFR). G-CSF stimulates myeloid precursor proliferation, differentiation, and survival through G-CSFR. The cytoplasmic portion of G-CSFR employs activation of Janus kinase and other downstream signaling pathways that lead to transcriptional changes that impact directly cell proliferation, differentiation and survival (Liongue et al. 2009). Though the mechanism is not clearly defined, it is believed that variants within this region of G-CSFR can shift the balance between these processes which may cause SCN and the leukemic events often seen with SCN (Germeshausen et al. 2007; Beekman et al. 2012).
In addition, acquired variants in CSF3R are strongly correlated to leukemic transformation seen with SCN (Germeshausen et al. 2007; Beekman et al. 2012; Skokowa et al. 2012; Dong et al. 1994; Dong et al. 1995; Tidow et al. 1997). Current data indicate CSF3R variants are not sufficient for development of leukemic transformation, but have been reported in 70% to 80% of SCN patients that developed AML or MDS (Dong et al. 1995; Germeshausen et al. 2007; Beekman et al. 2012; Skokowa et al. 2012). Variants in the CSF3R gene associated with progression to leukemia are located in the carboxy-terminal, intracellular portion of the G-CSFR protein and involve primarily truncating / loss of function changes that lead to enhanced proliferation, resistance to apoptosis, and increased cell survival (Germeshausen et al. 2007; McLemore et al. 1998; Hunter et al. 2000). In addition, an activating CSF3R gene variant defined as Thr617Asn has been reported in patients with neutrophilia (Plo et al. 2009).
Clinical Sensitivity - Sequencing with CNV PGxome
Variants in the CSF3R gene have been reported as the primary cause of disease in only a handful of SCN patients, but CSF3R variants have been reported in 70% to 80% of SCN patients who develop MDS / AML (Dong et al. 1995; Germeshausen et al. 2007; Beekman et al. 2012; Skokowa et al. 2012).
Reports of large deletions / duplications in the CSF3R gene are rare.
Testing Strategy
This test provides full coverage of all coding exons of the CSF3R gene plus 10 bases of flanking noncoding DNA in all available transcripts along with other non-coding regions in which pathogenic variants have been identified at PreventionGenetics or reported elsewhere. We define full coverage as >20X NGS reads or Sanger sequencing. PGnome panels typically provide slightly increased coverage over the PGxome equivalent. PGnome sequencing panels have the added benefit of additional analysis and reporting of deep intronic regions (where applicable).
Dependent on the sequencing backbone selected for this testing, discounted reflex testing to any other similar backbone-based test is available (i.e., PGxome panel to whole PGxome; PGnome panel to whole PGnome).
Indications for Test
Patients with recurring bacterial infections, a family history of SCN, or neutropenia unrelated to other known syndromes (e.g. Chediak-Higashi Syndrome, Hermansky Pudlak Syndrome, or Griscelli Syndrome) and who have evidence of full myeloid cell maturation in bone marrow. This test may also be considered for the reproductive partners of individuals who carry pathogenic variants in CSF3R.
Patients with recurring bacterial infections, a family history of SCN, or neutropenia unrelated to other known syndromes (e.g. Chediak-Higashi Syndrome, Hermansky Pudlak Syndrome, or Griscelli Syndrome) and who have evidence of full myeloid cell maturation in bone marrow. This test may also be considered for the reproductive partners of individuals who carry pathogenic variants in CSF3R.
Gene
Official Gene Symbol | OMIM ID |
---|---|
CSF3R | 138971 |
Inheritance | Abbreviation |
---|---|
Autosomal Dominant | AD |
Autosomal Recessive | AR |
X-Linked | XL |
Mitochondrial | MT |
Disease
Name | Inheritance | OMIM ID |
---|---|---|
Hereditary Neutrophilia | 162830 |
Citations 
- Ancliff PJ, Blundell MP, Cory GO, Calle Y, Worth A, Kempski H, Burns S, Jones GE, Sinclair J, Kinnon C, Hann IM, Gale RE, Linch DC, Thrasher AJ. 2006. Two novel activating mutations in the Wiskott-Aldrich syndrome protein result in congenital neutropenia. Blood 108: 2182–2189. PubMed ID: 16804117
- Beekman R, Valkhof MG, Sanders MA, Strien PMH van, Haanstra JR, Broeders L, Geertsma-Kleinekoort WM, Veerman AJP, Valk PJM, Verhaak RG, Löwenberg B, Touw IP. 2012. Sequential gain of mutations in severe congenital neutropenia progressing to acute myeloid leukemia. Blood 119: 5071–5077. PubMed ID: 22371884
- Bellanne-Chantelot C. 2004. Mutations in the ELA2 gene correlate with more severe expression of neutropenia: a study of 81 patients from the French Neutropenia Register. Blood 103: 4119–4125. PubMed ID: 14962902
- Boxer LA, Newburger PE. 2007. A molecular classification of congenital neutropenia syndromes. Pediatric Blood & Cancer 49: 609–614. PubMed ID: 17584878
- Boztug K, Appaswamy G, Ashikov A, Schäffer AA, Salzer U, Diestelhorst J, Germeshausen M, Brandes G, Lee-Gossler J, Noyan F. 2009. A syndrome with congenital neutropenia and mutations in G6PC3. New England Journal of Medicine 360: 32–43. PubMed ID: 19118303
- Dong F, Brynes RK, Tidow N, Welte K, Löwenberg B, Touw IP. 1995. Mutations in the gene for the granulocyte colony-stimulating–factor receptor in patients with acute myeloid leukemia preceded by severe congenital neutropenia. New England Journal of Medicine 333: 487–493. PubMed ID: 7542747
- Dong F, Hoefsloot LH, Schelen AM, Broeders CA, Meijer Y, Veerman AJ, Touw IP, Löwenberg B. 1994. Identification of a nonsense mutation in the granulocyte-colony-stimulating factor receptor in severe congenital neutropenia. Proc Natl Acad Sci U S A 91: 4480–4484. PubMed ID: 7514305
- Freedman MH, Bonilla MA, Fier C, Bolyard AA, Scarlata D, Boxer LA, Brown S, Cham B, Kannourakis G, Kinsey SE. 2000. Myelodysplasia syndrome and acute myeloid leukemia in patients with congenital neutropenia receiving G-CSF therapy. Blood 96: 429–436. PubMed ID: 10887102
- Germeshausen M, Ballmaier M, Welte K. 2007. Incidence of CSF3R mutations in severe congenital neutropenia and relevance for leukemogenesis: results of a long-term survey. Blood 109: 93–99. PubMed ID: 16985178
- Gilman PA, Jackson DP, Guild HG. 1970. Congenital agranulocytosis: prolonged survival and terminal acute leukemia. Blood 36: 576–585. PubMed ID: 4319697
- Hunter MG, Avalos BR. 2000. Granulocyte colony-stimulating factor receptor mutations in severe congenital neutropenia transforming to acute myelogenous leukemia confer resistance to apoptosis and enhance cell survival. Blood 95: 2132–2137. PubMed ID: 10706885
- Klein C. 2011. Genetic defects in severe congenital neutropenia: emerging insights into life and death of human neutrophil granulocytes. Annu. Rev. Immunol. 29: 399–413. PubMed ID: 21219176
- Kostman R. 1975. Infantile genetic agranulocytosis. A review with presentation of ten new cases. Acta Paediatr Scand 64: 362–368. PubMed ID: 1130195
- Liongue C, Wright C, Russell AP, Ward AC. 2009. Granulocyte colony-stimulating factor receptor: Stimulating granulopoiesis and much more. The International Journal of Biochemistry & Cell Biology 41: 2372–2375. PubMed ID: 19699815
- McLemore ML, Poursine-Laurent J, Link DC. 1998. Increased granulocyte colony-stimulating factor responsiveness but normal resting granulopoiesis in mice carrying a targeted granulocyte colony-stimulating factor receptor mutation derived from a patient with severe congenital neutropenia. Journal of Clinical Investigation 102: 483. PubMed ID: 9691084
- Pasquet M, Bellanné-Chantelot C, Tavitian S, Prade N, Beaupain B, LaRochelle O, Petit A, Rohrlich P, Ferrand C, Den Neste E Van, Poirel HA, Lamy T, Ouachée-Chardin M, Mansat-De Mas V, Corre J, Récher C, Plat G, Bachelerie F, Donadieu J, Delabesse E. 2013. High frequency of GATA2 mutations in patients with mild chronic neutropenia evolving to MonoMac syndrome, myelodysplasia, and acute myeloid leukemia. Blood 121: 822–829. PubMed ID: 23223431
- Person RE, Li F-Q, Duan Z, Benson KF, Wechsler J, Papadaki HA, Eliopoulos G, Kaufman C, Bertolone SJ, Nakamoto B, Papayannopoulou T, Grimes HL, Horwitz M. 2003. Mutations in proto-oncogene GFI1 cause human neutropenia and target ELA2. Nat. Genet. 34: 308–312. PubMed ID: 12778173
- Plo I, Zhang Y, Couédic J-PL, Nakatake M, Boulet J-M, Itaya M, Smith SO, Debili N, Constantinescu SN, Vainchenker W, Louache F, Botton S de. 2009. An activating mutation in the CSF3R gene induces a hereditary chronic neutrophilia. J Exp Med 206: 1701–1707. PubMed ID: 19620628
- Rosenberg PS, Zeidler C, Bolyard AA, Alter BP, Bonilla MA, Boxer LA, Dror Y, Kinsey S, Link DC, Newburger PE, Shimamura A, Welte K, Dale DC. 2010. Stable Long-Term Risk of Leukaemia in Patients with Severe Congenital Neutropenia Maintained on G-CSF Therapy. Br J Haematol 150: 196-199. PubMed ID: 20456363
- Rosenberg PS. 2006. The incidence of leukemia and mortality from sepsis in patients with severe congenital neutropenia receiving long-term G-CSF therapy. Blood 107: 4628–4635. PubMed ID: 16497969
- Skokowa J, Steinemann D, Katsman-Kuipers JE, Zeidler C, Klimenkova O, Klimiankou M, Ünalan M, Kandabarau S, Makaryan V, Beekman R, Behrens K, Stocking C, et al. 2014. Cooperativity of RUNX1 and CSF3R mutations in severe congenital neutropenia: a unique pathway in myeloid leukemogenesis. Blood 123: 2229–2237. PubMed ID: 24523240
- Stepensky P, Saada A, Cowan M, Tabib A, Fischer U, Berkun Y, Saleh H, Simanovsky N, Kogot-Levin A, Weintraub M, Ganaiem H, Shaag A, Zenvirt S, Borkhardt A, Elpeleg O, Bryant NJ, Mevorach D. 2013. The Thr224Asn mutation in the VPS45 gene is associated with the congenital neutropenia and primary myelofibrosis of infancy. Blood 121: 5078–5087. PubMed ID: 23599270
- Tidow N, Pilz C, Teichmann B, Müller-Brechlin A, Germeshausen M, Kasper B, Rauprich P, Sykora K-W, Welte K. 1997. Clinical relevance of point mutations in the cytoplasmic domain of the granulocyte colony-stimulating factor receptor gene in patients with severe congenital neutropenia. Blood 89: 2369–2375. PubMed ID: 9116280
- Triot A, Järvinen PM, Arostegui JI, Murugan D, Kohistani N, Díaz JLD, Racek T, Puchałka J, Gertz EM, Schäffer AA, Kotlarz D, Pfeifer D, et al. 2014. Inherited biallelic CSF3R mutations in severe congenital neutropenia. Blood 123: 3811–3817. PubMed ID: 24753537
- Xia J, Bolyard AA, Rodger E, Stein S, Aprikyan AA, Dale DC, Link DC. 2009. Prevalence of mutations in ELANE , GFI1 , HAX1 , SBDS , WAS and G6PC3 in patients with severe congenital neutropenia. British Journal of Haematology 147: 535–542. PubMed ID: 19775295
- Xia J, Link DC. 2008. Severe congenital neutropenia and the unfolded protein response. Curr. Opin. Hematol. 15: 1–7. PubMed ID: 18043239
Ordering/Specimens 
Ordering Options
We offer several options when ordering sequencing tests. For more information on these options, see our Ordering Instructions page. To view available options, click on the Order Options button within the test description.
myPrevent - Online Ordering
- The test can be added to your online orders in the Summary and Pricing section.
- Once the test has been added log in to myPrevent to fill out an online requisition form.
- PGnome sequencing panels can be ordered via the myPrevent portal only at this time.
Requisition Form
- A completed requisition form must accompany all specimens.
- Billing information along with specimen and shipping instructions are within the requisition form.
- All testing must be ordered by a qualified healthcare provider.
For Requisition Forms, visit our Forms page
If ordering a Duo or Trio test, the proband and all comparator samples are required to initiate testing. If we do not receive all required samples for the test ordered within 21 days, we will convert the order to the most effective testing strategy with the samples available. Prior authorization and/or billing in place may be impacted by a change in test code.
Specimen Types
Specimen Requirements and Shipping Details
PGxome (Exome) Sequencing Panel
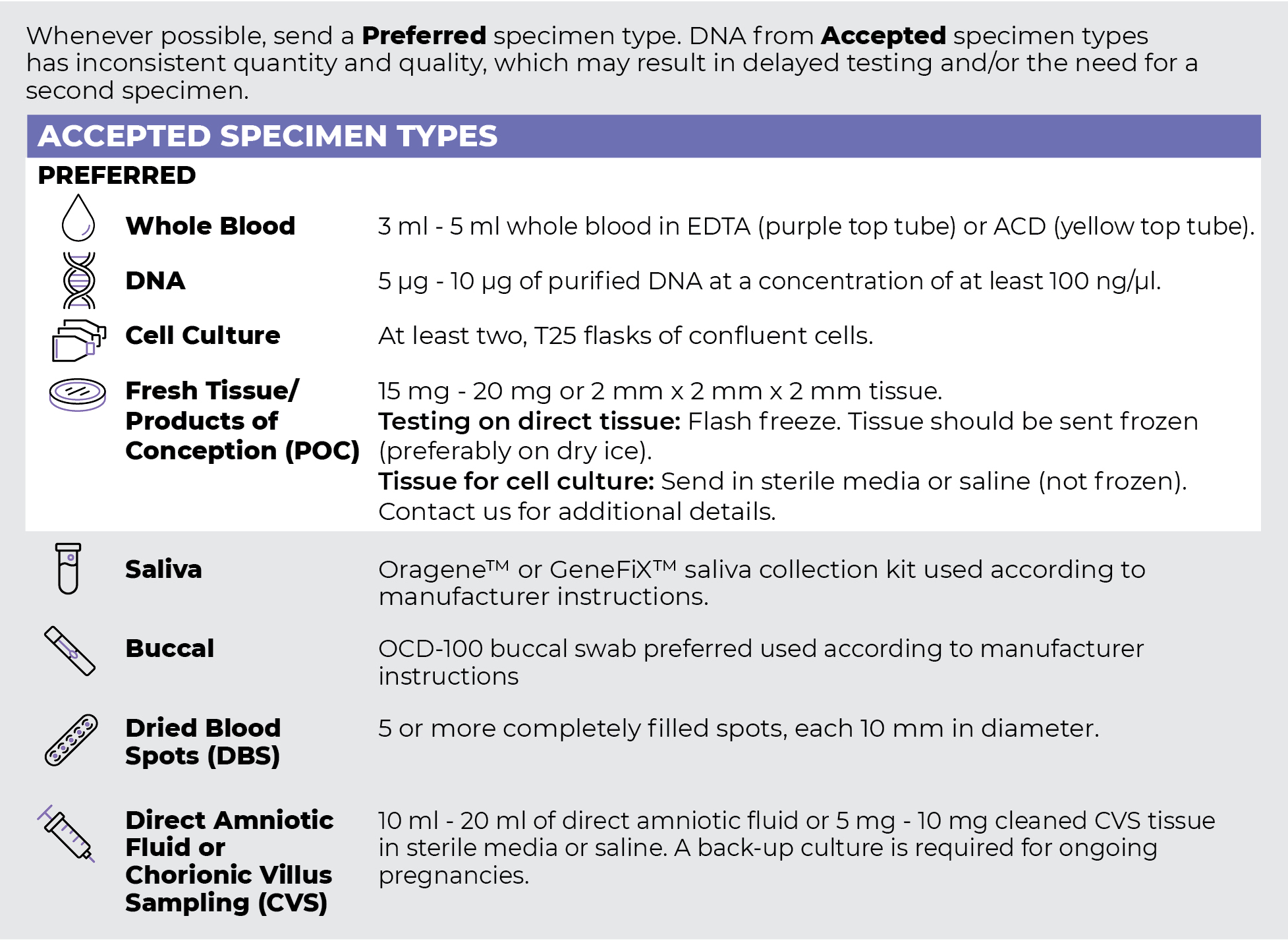
PGnome (Genome) Sequencing Panel
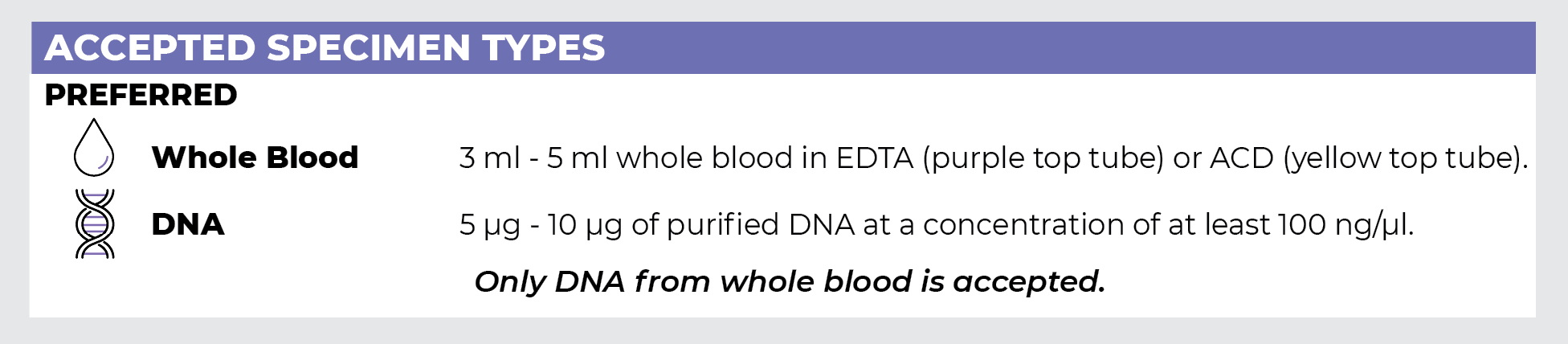
ORDER OPTIONS
View Ordering Instructions1) Select Test Type
2) Select Additional Test Options
No Additional Test Options are available for this test.