PTPN11-Related Disorders via the PTPN11 Gene
Summary and Pricing 
Test Method
Sequencing and CNV Detection via NextGen Sequencing using PG-Select Capture ProbesTest Code | Test Copy Genes | Test CPT Code | Gene CPT Codes Copy CPT Code | Base Price | |
---|---|---|---|---|---|
8179 | PTPN11 | 81406 | 81406,81479 | $990 | Order Options and Pricing |
Pricing Comments
Testing run on PG-select capture probes includes CNV analysis for the gene(s) on the panel but does not permit the optional add on of exome-wide CNV analysis. Any of the NGS platforms allow reflex to other clinically relevant genes, up to whole exome or whole genome sequencing depending upon the base platform selected for the initial test.
An additional 25% charge will be applied to STAT orders. STAT orders are prioritized throughout the testing process.
This test is also offered via a custom panel (click here) on our exome or genome backbone which permits the optional add on of exome-wide CNV or genome-wide SV analysis.
Turnaround Time
3 weeks on average for standard orders or 2 weeks on average for STAT orders.
Please note: Once the testing process begins, an Estimated Report Date (ERD) range will be displayed in the portal. This is the most accurate prediction of when your report will be complete and may differ from the average TAT published on our website. About 85% of our tests will be reported within or before the ERD range. We will notify you of significant delays or holds which will impact the ERD. Learn more about turnaround times here.
Targeted Testing
For ordering sequencing of targeted known variants, go to our Targeted Variants page.
Clinical Features and Genetics 
Clinical Features
Noonan syndrome (NS, OMIM 163950) is a relatively common developmental disorder that is characterized by dysmorphic facial features, growth and congenital heart defects, and musculoskeletal abnormalities. Cardiac abnormalities are found in up to 80% of patients and include pulmonary valve stenosis, atrial septal defect, atrioventricular canal defect, and hypertrophic cardiomyopathy. Musculoskeletal abnormalities include short stature, chest deformity with sunken or raised sternum, and short webbed neck. Several additional abnormalities have been described and include renal, genital, hematological, neurologic, cognitive, behavioral, gastrointestinal, dental, and lymphatic findings. Intelligence is usually normal; however, learning disabilities may be present. NS is characterized by an extensive clinical heterogeneity, even among members of the same family. Diagnosis is often made in infancy or early childhood. Symptoms often change and lessen with advancing age. Infants with NS are at risk of developing juvenile myelomonocytic leukemia (JMML OMIM 607785). The prevalence of NS is estimated at 1 in 1000-2500 births worldwide (Allanson et al. Am J Med Genet 21:507-514, 1985; Romano et al. Pediatrics 126:746-759, 2010).
LEOPARD syndrome (multiple lentigines, electrocardiographic-conduction abnormalities, ocular hypertelorism, pulmonary stenosis, abnormal genitalia, retardation of growth, sensorineural deafness, OMIM 151100) is a rare congenital developmental disorder characterized by skin pigmentation anomalies including multiple lentigines and café au lait spots, hypertrophic cardiomyopathy, pulmonary valve stenosis, and deafness. Other less common features include short stature, mild mental retardation, and abnormal genitalia (Legius et al. J Med Genet 39:571-574, 2002; Sarkozy et al. J Med Genet 41:e68, 2004; see also Gelb and Tartaglia, GeneReviews, 2010).
Metachondromatosis (MC OMIM 156250) is a rare skeletal dysplasia characterized by multiple exostoses mainly in the hands and feet. Additional features include enchondromas of the iliac crest and metaphyseal region of the long bones of the lower extremities and periarticular calcifications. The exostoses may result in peroneal-nerve symptoms, which are relieved by surgery. The original exostoses may regress spontaneously with age, while new ones may develop. Although skeletal radiological findings of MC resemble those of multiple enchondromatosis (OMIM 166000), the two conditions can be easily distinguished. In MC, the exostoses point toward the nearby joints. In addition, they do not result in shortening of affected long bones, bowing, joint deformity, or joint dislocation, as is the case in multiple enchondromatosis (Kennedy Radiology 48:117-118, 1983; Bassett et al. J Bone Joint Surg Am 67:811-814, 1985).
Genetics
Noonan syndrome (NS) is caused by gain of function variants in various genes within the RAS/MAPK pathway, including PTPN11. These variants appear to activate the gene product (SHP2 protein). To date, seven RAS/MAPK genes (PTPN11, SOS1, RAF1, KRAS, SHOC2, BRAF, and NRAS) have been associated with NS. PTPN11 variants are the most common cause of NS, and account for ~ 50% of all cases genotyped (Allanson and Roberts, GeneReviews, 2011). Over 70 variants were reported. Most causative variants are missense. A few small deletion or insertion variants have been reported (Tartaglia et al. Am J Hum Genet 78:279-290, 2006). Except for two large genomic duplications that included the entire PTPN11 gene (Shchelochkov et al. Am J Med Genet 146A:1042-1048, 2008; Graham et al. Am J Med Genet 149A:2122-2128, 2009), no gross defects or rearrangements in PTPN11 have been reported in patients with NS. Although de novo variants are found in a substantial fraction of patients, familial cases have been reported. In these families, NS is inherited in an autosomal dominant manner with variable expressivity (Romano et al. Pediatrics 126:746-759, 2010).
Genotype-phenotype correlations are slowly being made. In particular, several groups have shown that pulmonary stenosis is more common in NS patients with PTPN11 variants, while hypertrophic cardiomyopathy is more common in patients without PTPN11 variants (Romano et al. 2010).
LEOPARD syndrome is caused by defects in three genes within the RAS/MAPK: PTPN11, RAF1 and BRAF (Digilio et al. Am J Hum Genet 71:389-394, 2002; Pandit et al. Nat Genet 39:1007-1012, 2007; Sarkozy et al. Hum Mutat 30:695-702, 2009). Unlike NS, LEOPARD syndrome variants act through a dominant negative effect, which appears to disrupt the function of the wild-type gene product (SHP2 protein) (Jopling et al. PLOS Genetics 3:e225, 2007). Ten different PTPN11 variants, all missense, have been reported in patients with LEOPARD. PTPN11 variants are the most common cause of LEOPARD syndrome, and account for over 90% of all cases genotyped. Parents of patients with LEOPARD syndrome are often asymptomatic, and de novo variants are common. However, familial cases have been reported. In these families, affected relatives are diagnosed only after the birth of a visibly affected child, and the disease is transmitted in an autosomal dominant manner with variable penetrance and expressivity (Gelb and Tartaglia, GeneReviews, 2010). Genotype-phenotype correlations have been proposed (see for example Limongelli et al. Am J Med Genet A 146:620-628, 2008).
Metachondromatosis (MC) is caused by loss-of-function variants in PTPN11. Recently, 13 heterozygous PTPN11 variants were reported. At this time, PTPN11 variants are the only known cause of MC. Variants were found in ~ 60% if the MC families that have been studied, suggesting that PTPN11 variants are a common cause of the disorder and that MC is genetically heterogeneous (Sobreira et al. PLoS Genet 6:e1000991, 2010; Bowen et al. PLoS Genet 7:e1002050, 2011). Variants are distributed along the entire coding region of the gene and include missense, splicing, small deletions, and complex rearrangements. MC is inherited in an autosomal dominant manner with incomplete penetrance.
Somatic PTPN11 variants account for ~ 34% of non-syndromic juvenile myelomonocytic leukemia (JMML) (Tartaglia et al. Nat Genet 34:148-150, 2003).
Clinical Sensitivity - Sequencing with CNV PG-Select
This test will detect causative variants in ~50% of NS patients, ~90% of LS patients, and ~60% of MC patients.
Testing Strategy
This test provides full coverage of all coding exons of the PTPN11 gene, plus ~10 bases of flanking noncoding DNA. We define full coverage as >20X NGS reads or Sanger sequencing.
Indications for Test
Patients with clinical features of NS, LEOPARD syndrome, and MC and their family members are candidates for this test. As the clinical features of cardio-facio-cutaneous syndrome and Costello syndrome overlap with NS, patients who test negative for variants in the genes most commonly associated with those conditions are also candidates.
Patients with clinical features of NS, LEOPARD syndrome, and MC and their family members are candidates for this test. As the clinical features of cardio-facio-cutaneous syndrome and Costello syndrome overlap with NS, patients who test negative for variants in the genes most commonly associated with those conditions are also candidates.
Gene
Official Gene Symbol | OMIM ID |
---|---|
PTPN11 | 176876 |
Inheritance | Abbreviation |
---|---|
Autosomal Dominant | AD |
Autosomal Recessive | AR |
X-Linked | XL |
Mitochondrial | MT |
Diseases
Name | Inheritance | OMIM ID |
---|---|---|
LEOPARD Syndrome | AD | 151100 |
Metachondromatosis | AD | 156250 |
Noonan Syndrome 1 | AD | 163950 |
Related Test
Name |
---|
Comprehensive Arrhythmia and Cardiomyopathy Panel |
Citations 
- Allanson JE, Hall JG, Hughes HE, Preus M, Witt RD. 1985. Noonan syndrome: the changing phenotype. Am. J. Med. Genet. 21: 507-514. PubMed ID: 4025385
- Allanson, Judith E MD , Roberts, Amy E MD. (2011). "Noonan Syndrome." PubMed ID: 20301303
- Bassett, G. S., Cowell, H. R. (1985). "Metachondromatosis. Report of four cases." J Bone Joint Surg Am 67(5): 811-4. PubMed ID: 3873457
- Bowen, M. E., et.al. (2011). "Loss-of-function mutations in PTPN11 cause metachondromatosis, but not Ollier disease or Maffucci syndrome." PLoS Genet 7(4): e1002050. PubMed ID: 21533187
- Digilio MC, Conti E, Sarkozy A, Mingarelli R, Dottorini T, Marino B, Pizzuti A, Dallapiccola B. 2002. Grouping of Multiple-Lentigines/LEOPARD and Noonan Syndromes on the PTPN11 Gene. The American Journal of Human Genetics 71: 389–394. PubMed ID: 12058348
- Gelb BD, Tartaglia M. 2010. LEOPARD Syndrome. In: Pagon RA, Adam MP, Ardinger HH, Bird TD, Dolan CR, Fong C-T, Smith RJ, and Stephens K, editors. GeneReviews(®), Seattle (WA): University of Washington, Seattle. PubMed ID: 20301557
- Graham JM, Kramer N, Bejjani BA, Thiel CT, Carta C, Neri G, Tartaglia M, Zenker M. 2009. Genomic duplication of PTPN11 is an uncommon cause of Noonan syndrome. American Journal of Medical Genetics Part A 149A: 2122–2128. PubMed ID: 19760651
- Kennedy. Metachondromatosis. Radiology 48:117-118, 1983 PubMed ID: 6602353
- Legius E, Schrander-Stumpel C, Schollen E, Pulles-Heintzberger C, Gewillig M, Fryns J-P. 2002. PTPN11 mutations in LEOPARD syndrome. J. Med. Genet. 39: 571–574. PubMed ID: 12161596
- Pandit B, Sarkozy A, Pennacchio LA, Carta C, Oishi K, Martinelli S, Pogna EA, Schackwitz W, Ustaszewska A, Landstrom A, Bos JM, Ommen SR, Esposito G, Lepri F, Faul C, Mundel P, López Siguero JP, Tenconi R, Selicorni A, Rossi C, Mazzanti L, Torrente I, Marino B, Digilio MC, Zampino G, Ackerman MJ, Dallapiccola B, Tartaglia M, Gelb BD. 2007. Gain-of-function RAF1 mutations cause Noonan and LEOPARD syndromes with hypertrophic cardiomyopathy. Nature Genetics 39: 1007–1012. PubMed ID: 17603483
- Romano AA, Allanson JE, Dahlgren J, Gelb BD, Hall B, Pierpont ME, Roberts AE, Robinson W, Takemoto CM, Noonan JA. 2010. Noonan syndrome: clinical features, diagnosis, and management guidelines. Pediatrics 126: 746-759. PubMed ID: 20876176
- Sarkozy A, Conti E, Digilio MC, Marino B, Morini E, Pacileo G, Wilson M, Calabrò R, Pizzuti A, Dallapiccola B. 2004. Clinical and molecular analysis of 30 patients with multiple lentigines LEOPARD syndrome. Journal of Medical Genetics 41: e68–e68. PubMed ID: 15121796
- Shchelochkov OA, Patel A, Weissenberger GM, Chinault AC, Wiszniewska J, Fernandes PH, Eng C, Kukolich MK, Sutton VR. 2008. Duplication of chromosome band 12q24.11q24.23 results in apparent Noonan syndrome. Am. J. Med. Genet. A 146A: 1042–1048. PubMed ID: 18348260
- Sobreira, N. L., et.al. (2009). "Whole-genome sequencing of a single proband together with linkage analysis identifies a Mendelian disease gene." PLoS Genet 6(6): e1000991. PubMed ID: 20577567
- Tartaglia M, Niemeyer CM, Fragale A, Song X, Buechner J, Jung A, Hählen K, Hasle H, Licht JD, Gelb BD. 2003. Somatic mutations in PTPN11 in juvenile myelomonocytic leukemia, myelodysplastic syndromes and acute myeloid leukemia. Nature genetics 34: 148–150. PubMed ID: 12717436
- Tartaglia, M., et.al. (2006). "Diversity and functional consequences of germline and somatic PTPN11 mutations in human disease." Am J Hum Genet 78(2): 279-90. PubMed ID: 16358218
Ordering/Specimens 
Ordering Options
We offer several options when ordering sequencing tests. For more information on these options, see our Ordering Instructions page. To view available options, click on the Order Options button within the test description.
myPrevent - Online Ordering
- The test can be added to your online orders in the Summary and Pricing section.
- Once the test has been added log in to myPrevent to fill out an online requisition form.
- PGnome sequencing panels can be ordered via the myPrevent portal only at this time.
Requisition Form
- A completed requisition form must accompany all specimens.
- Billing information along with specimen and shipping instructions are within the requisition form.
- All testing must be ordered by a qualified healthcare provider.
For Requisition Forms, visit our Forms page
If ordering a Duo or Trio test, the proband and all comparator samples are required to initiate testing. If we do not receive all required samples for the test ordered within 21 days, we will convert the order to the most effective testing strategy with the samples available. Prior authorization and/or billing in place may be impacted by a change in test code.
Specimen Types
Specimen Requirements and Shipping Details
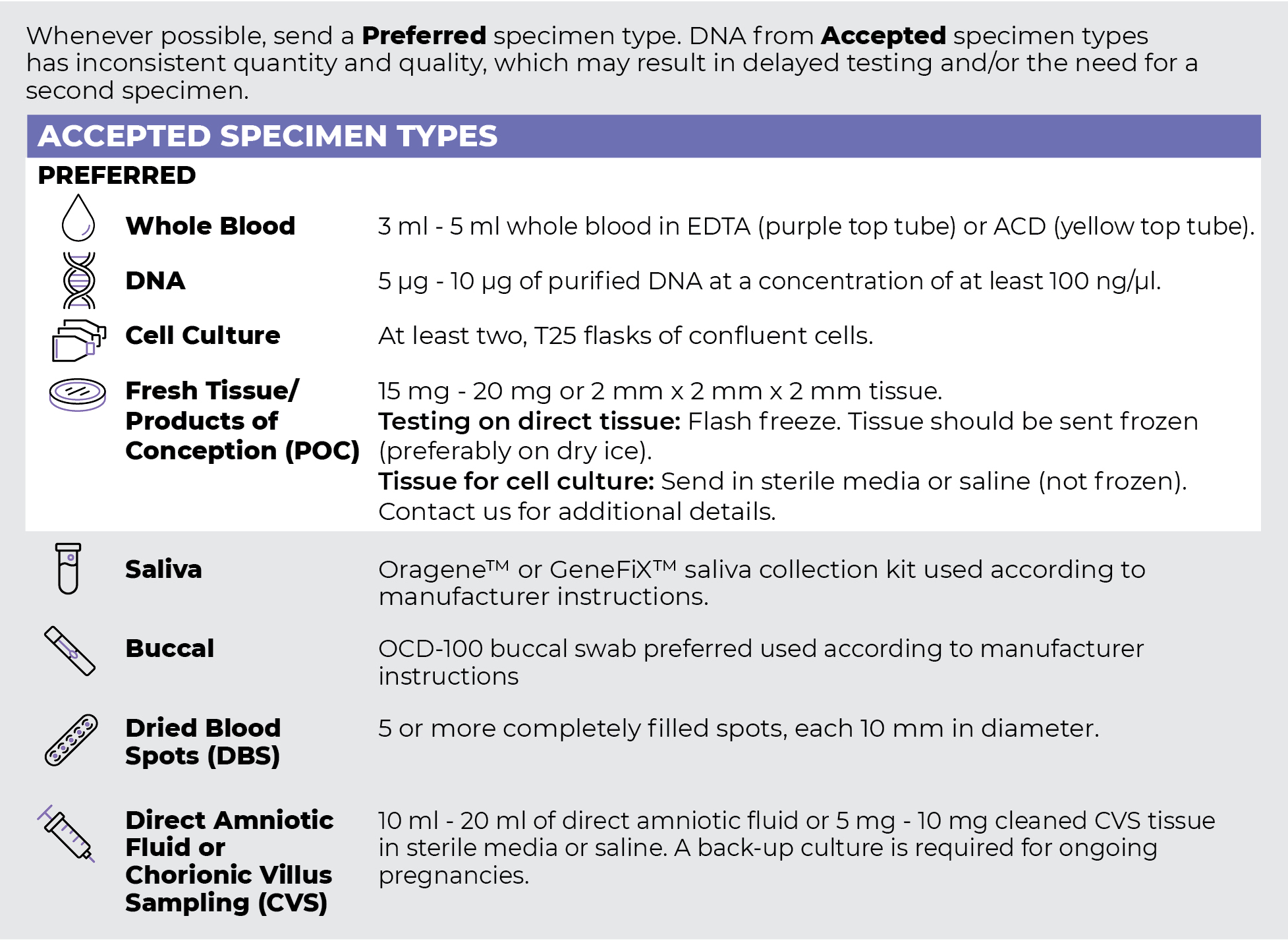
ORDER OPTIONS
View Ordering Instructions1) Select Test Type
2) Select Additional Test Options
No Additional Test Options are available for this test.