Hypercalcemic and Hypocalcemic Disorders via the CASR Gene
Summary and Pricing 
Test Method
Sequencing and CNV Detection via NextGen Sequencing using PG-Select Capture ProbesTest Code | Test Copy Genes | Test CPT Code | Gene CPT Codes Copy CPT Code | Base Price | |
---|---|---|---|---|---|
4115 | CASR | 81405 | 81405,81479 | $990 | Order Options and Pricing |
Pricing Comments
Testing run on PG-select capture probes includes CNV analysis for the gene(s) on the panel but does not permit the optional add on of exome-wide CNV analysis. Any of the NGS platforms allow reflex to other clinically relevant genes, up to whole exome or whole genome sequencing depending upon the base platform selected for the initial test.
An additional 25% charge will be applied to STAT orders. STAT orders are prioritized throughout the testing process.
This test is also offered via a custom panel (click here) on our exome or genome backbone which permits the optional add on of exome-wide CNV or genome-wide SV analysis.
Turnaround Time
3 weeks on average for standard orders or 2 weeks on average for STAT orders.
Please note: Once the testing process begins, an Estimated Report Date (ERD) range will be displayed in the portal. This is the most accurate prediction of when your report will be complete and may differ from the average TAT published on our website. About 85% of our tests will be reported within or before the ERD range. We will notify you of significant delays or holds which will impact the ERD. Learn more about turnaround times here.
Targeted Testing
For ordering sequencing of targeted known variants, go to our Targeted Variants page.
Clinical Features and Genetics 
Clinical Features
Defects of the calcium-sensing receptor (CaSR) can cause both hypercalcemic and hypocalcemic disorders (Hannan et al. Best Pract Res Clin Endocrinol Metab 27(3):359-71, 2013; Egbuna et al. Best Pract Res Clin Rheumatol 22(1):129-48, 2008). Loss-of-function (inactivating) mutations of its encoding gene CASR lead to three hypercalcemic disorders: familial hypocalciuric hypercalcemia (FHH), neonatal severe hyperparathyroidism (NSHPT) and primary hyperparathyroidism (PHPT). On the other hand, gain-of-function (activating) CASR mutations have been associated with two hypocalcemic disorders: autosomal dominant hypocalcemia (ADH) and Bartter syndrome type V.
Familial hypocalciuric hypercalcemia (FHH) is a heritable disorder of mineral homeostasis characterized by lifelong elevation of serum calcium concentrations. FHH patients are usually asymptomatic, and the disorder is generally considered benign. Clinical features of FHH include hypermagnesemia and low urinary calcium excretion. FHH patients have normal or mildly elevated circulating parathyroid hormone (PTH) levels. In uncommon symptomatic cases, adult patients have chondrocalcinosis and pancreatitis while some children may develop neonatal severe hyperparathyroidism (NSHPT). FHH is a genetically heterogeneous disorder and consists of three variants: FHH1, FHH2 and FHH3. FHH1 (OMIM# 145980) is caused by dominant loss-of-function CASR mutations. The age of FHH onset is mostly in infancy but severe FHH can present in either childhood or early adulthood.
Neonatal severe hyperparathyroidism (NSHPT; OMIM# 239200) is a life-threatening disorder characterized by severe hypercalcaemia, hyperparathyroidism, enlarged parathyroid glands, hypotonia, bone demineralization, fractures, ribcage deformities, respiratory distress and failure to thrive. NSHPT usually manifests in the first 6 months of life. Early diagnosis of NSHPT is critical because usually a parathyroidectomy is required. Severity of disease manifestations can vary within the same family.
Within a variable phenotypic spectrum of hypercalcemia associated with loss-of-function CASR mutations, primary hyperparathyroidism (PHPT) is comparable to FHH with overlapping features but represents a distinct clinical entity. The age of PHPT onset is in adulthood. The severity of hypercalcemia in PHPT patients is variable. In addition to hypercalcemia, PHPT patients may have non-suppressed urinary calcium excretion, elevated serum PTH concentrations, kidney stones, and/or osteoporosis. Parathyroid adenomas or hyperplasia are also common.
Autosomal dominant hypocalcemia (ADH; OMIM# 601198) is characterized by mild to moderate hypocalcemia. ADH patients are generally asymptomatic, but some patients may have neuromuscular symptoms such as paresthesiae, carpopedal spasm and seizures. Other common features of ADH include hyperphosphatemia, hypomagnesemia and relative hypercalciuria.
Bartter syndrome is a clinically and genetically heterogeneous group of electrolyte disorders characterized by renal salt wasting, which results in volume depletion with consequent hyperreninemic hyperaldosteronism and hypokalemic alkalosis. Bartter syndrome type V is caused by activating CASR mutations.
Genetics
CASR has 6 coding exons that encode the calcium-sensing receptor, a G-protein-coupled receptor (GPCR), which is essential in extracellular calcium homeostasis and regulation of salt-water metabolism (Pollak et al. Cell 75(7):1297-303, 1993; Hannan et al., 2013). Genetic defects located throughout the CASR gene include missense, nonsense, splicing site mutations, and small deletion/insertions, while large deletions and insertions are very rare (Human Gene Mutation Database). The majority (>50%) of mutations associated with hypercalcemic and hypocalcemic disorders are located in the extracellular domain (ECD) of CaSR (Hannan et al. Hum Mol Genet 21(12):2768-2778, 2012).
Familial hypocalciuric hypercalcemia type 1 (FHH1) is inherited in an autosomal dominant manner and is caused by heterozygous CASR mutations (Pollak et al., 1993). FHH-associated CASR mutations account for approximately 50% of all documented CASR mutations (Human Gene Mutation Database; Hannan et al., 2013). These FHH-associated mutations are predominantly missense substitutions but other type mutations have also been reported. Compared to missense substitutions, truncating mutations are associated with a milder hypercalcemic phenotype, suggesting a dominant-negative effect of missense substitutions. Notably, the majority of FHH-associated mutations are located within the extracellular domain (ECD) of CaSR and clustered at negatively charged regions. CASR mutations are detected in only around 65% of FHH patients; the other two recently identified FHH genes are AP2S1 (FHH3) and GNA11 (FHH2) (Nesbit et al. Nat Genet 45(1):93-97, 2013; Nesbit et al. N Engl J Med 368(26):2476-2486, 2013).
Neonatal severe hyperparathyroidism (NSHPT) is an autosomal recessive disorder caused by familial homozygous or compound heterozygous loss-of-function CASR mutations (Pollak et al., 1993). Interestingly, dominant-acting heterozygous CASR mutations have also been reported to arise de novo in sporadic NSHPT patients with milder phenotypes (Pearce et al. J Clin Invest 96(6):2683-2692, 1995; Bai et al. J Clin Invest 99(1):88-96, 1997). Approximately 10% of documented CASR mutations are associated with NSHPT, more than 40% of which are truncating mutations (either nonsense or frameshift) (Human Gene Mutation Database; Hannan et al., 2013).
Primary hyperparathyroidism (PHPT) is caused by either heterozygous or homozygous loss-of-function CASR mutations (Hannan et al. Clin Endocrinol. 73(6):715-22, 2010; Hannan et al., 2013). Hypercalcemic disorders after infancy, including PHPT and severe FHH, may be associated with the degree of function loss resulting from underlying CASR mutations. Unknown genetic modifiers could also contribute to the variability of these hypercalcemic phenotypes.
Autosomal dominant hypocalcemia (ADH) is caused by gain-of-function CASR mutations (Pollak et al. Nat Genet 8(3):303-307, 1994). Approximately 30% of documented CASR mutations are associated with ADH, 95% of which are missense mutations (Hannan et al., 2013). There are two hotspots of ADH-associated CASR mutations: the second peptide loop of the ECD (residues 116–136), and a region that encompasses transmembrane domains 6 and 7 and the intervening third extracellular loop (residues 819–837). The other recently identified ADH gene is GNA11 (Nesbit et al., 2013).
Bartter syndrome type V is an autosomal dominant disorder caused by activating gain-of-function CASR mutations (Vargas-Poussou et al. J Am Soc Nephrol 13(9):2259-2266, 2002; Watanabe et al. Lancet 360(9334):692-694, 2002; Vezzoli et al. J Nephrol 19(4):525-528, 2006). To date, only four CASR mutations (K29E, L125P, C131W and A843E) have been found in Bartter syndrome type V.
Clinical Sensitivity - Sequencing with CNV PG-Select
CASR mutations are detected in around 65% of FHH patients (Hannan et al. Best Pract Res Clin Endocrinol Metab 27(3):359-371, 2013). In a cohort of 294 patients with clinical features suggestive of hypercalcemic (FHH and NSHPT) or hypocalcemic (ADH) disorders, the overall CASR mutation detection rate is 29% (26% in FHH and NSHPT; 41% in ADH) (Hannan et al. Hum Mol Genet 21(12):2768-2778, 2012). CASR mutation detection rate in a large cohort of patients with PTPH or Bartter syndrome type V is unknown because these CASR mutations have been only reported in limited individual cases.
Testing Strategy
This test provides full coverage of all coding exons of the CASR gene, plus ~10 bases of flanking noncoding DNA. We define full coverage as >20X NGS reads or Sanger sequencing.
Indications for Test
Candidates for this test are patients with any of the hypercalcemic or hypocalcemic disorders listed. Testing is also indicated for family members of patients who have known CASR mutations. This test may also be considered for the reproductive partners of individuals who carry pathogenic variants in CASR.
Candidates for this test are patients with any of the hypercalcemic or hypocalcemic disorders listed. Testing is also indicated for family members of patients who have known CASR mutations. This test may also be considered for the reproductive partners of individuals who carry pathogenic variants in CASR.
Gene
Official Gene Symbol | OMIM ID |
---|---|
CASR | 601199 |
Inheritance | Abbreviation |
---|---|
Autosomal Dominant | AD |
Autosomal Recessive | AR |
X-Linked | XL |
Mitochondrial | MT |
Diseases
Name | Inheritance | OMIM ID |
---|---|---|
Familial Benign Hypercalcemia | AD | 145980 |
Hyperparathyroidism, Neonatal Severe Primary | AR, AD | 239200 |
Hypocalcemia, autosomal dominant | AD | 601198 |
Related Tests
Name |
---|
Chronic Pancreatitis Panel |
Familial Hypocalciuric Hypercalcemia (FHH) Panel |
Hypomagnesemia Panel |
Hypoparathyroidism Panel |
Nephrolithiasis and Nephrocalcinosis Panel |
Citations 
- Bai, M. et al. (1997). "In vivo and in vitro characterization of neonatal hyperparathyroidism resulting from a de novo, heterozygous mutation in the Ca2+-sensing receptor gene: normal maternal calcium homeostasis as a cause of secondary hyperparathyroidism in familial benign hypocalciuric hypercalcemia." J Clin Invest 99(1):88-96. PubMed ID: 9011580
- Egbuna, O. et al. (2008). "Hypercalcaemic and hypocalcaemic conditions due to calcium-sensing receptor mutations." Best Pract Res Clin Rheumatol 22(1):129-148. PubMed ID: 18328986
- Hannan, F. et al. (2010). “A homozygous inactivating calcium-sensing receptor mutation, Pro339Thr, is associated with isolated primary hyperparathyroidism: correlation between location of mutations and severity of hypercalcaemia.” Clin Endocrinol 73(6):715-722. PubMed ID: 20846291
- Hannan, F. et al. (2012). " Identification of 70 calcium-sensing receptor mutations in hyper- and hypo-calcaemic patients: evidence for clustering of extracellular domain mutations at calcium-binding sites." Hum Mol Genet 21(12):2768-2778. PubMed ID: 22422767
- Hannan, F. et al. (2013). "Calcium-sensing receptor (CaSR) mutations and disorders of calcium, electrolyte and water metabolism." Best Pract Res Clin Endocrinol Metab 27(3):359-371. PubMed ID: 23856265
- Human Gene Mutation Database (Bio-base).
- Nesbit, M. et al. (2013). "Mutations in AP2S1 cause familial hypocalciuric hypercalcemia type 3." Nat Genet 45(1):93-97. PubMed ID: 23222959
- Nesbit, M. et al. (2013.) "Mutations affecting G-protein subunit α11 in hypercalcemia and hypocalcemia." N Engl J Med 368(26):2476-2486. PubMed ID: 23802516
- Pearce, S. et al. (1995). "Calcium-sensing receptor mutations in familial benign hypercalcemia and neonatal hyperparathyroidism." J Clin Invest 96(6):2683-2692. PubMed ID: 8675635
- Pollak, M. et al. (1993). "Mutations in the human Ca(2+)-sensing receptor gene cause familial hypocalciuric hypercalcemia and neonatal severe hyperparathyroidism." Cell 75(7):1297-1303. PubMed ID: 7916660
- Pollak, M. et al. (1994). "Autosomal dominant hypocalcaemia caused by a Ca(2+)-sensing receptor gene mutation." Nat Genet 8(3):303-307. PubMed ID: 7874174
- Vargas-Poussou, R. et al. (2002). “Functional characterization of a calcium-sensing receptor mutation in severe autosomal dominant hypocalcemia with a Bartter-like syndrome.” J Am Soc Nephrol 13(9):2259-2266. PubMed ID: 12191970
- Vezzoli, G. et al. (2006). "Autosomal dominant hypocalcemia with mild type 5 Bartter syndrome." J Nephrol 19(4):525-528. PubMed ID: 17048213
- Watanabe, S. et al. (2002). “Association between activating mutations of calcium-sensing receptor and Bartter's syndrome.” Lancet 360(9334):692-694. PubMed ID: 12241879
Ordering/Specimens 
Ordering Options
We offer several options when ordering sequencing tests. For more information on these options, see our Ordering Instructions page. To view available options, click on the Order Options button within the test description.
myPrevent - Online Ordering
- The test can be added to your online orders in the Summary and Pricing section.
- Once the test has been added log in to myPrevent to fill out an online requisition form.
- PGnome sequencing panels can be ordered via the myPrevent portal only at this time.
Requisition Form
- A completed requisition form must accompany all specimens.
- Billing information along with specimen and shipping instructions are within the requisition form.
- All testing must be ordered by a qualified healthcare provider.
For Requisition Forms, visit our Forms page
If ordering a Duo or Trio test, the proband and all comparator samples are required to initiate testing. If we do not receive all required samples for the test ordered within 21 days, we will convert the order to the most effective testing strategy with the samples available. Prior authorization and/or billing in place may be impacted by a change in test code.
Specimen Types
Specimen Requirements and Shipping Details
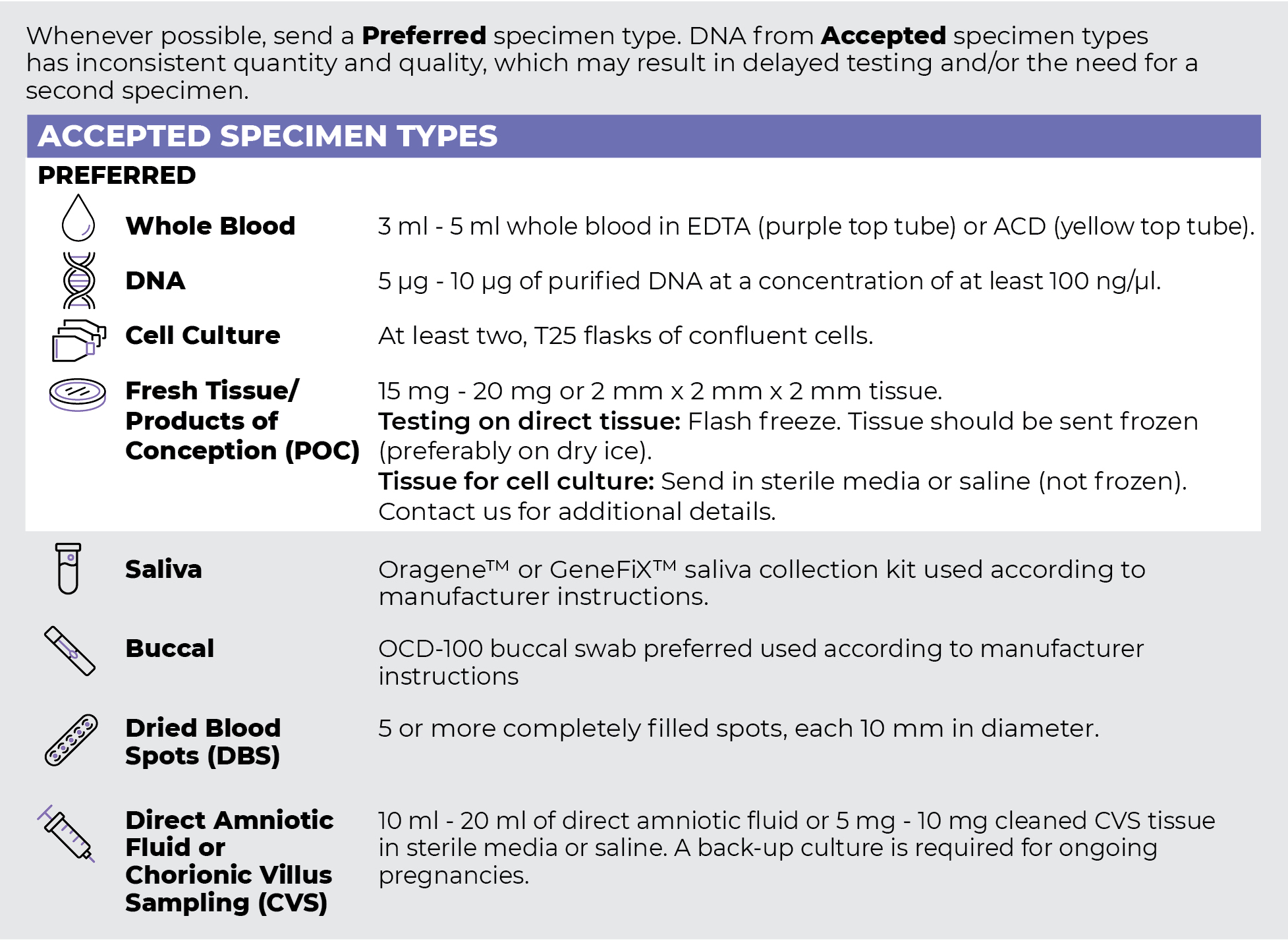
ORDER OPTIONS
View Ordering Instructions1) Select Test Type
2) Select Additional Test Options
No Additional Test Options are available for this test.