Chronic Pancreatitis Panel
Summary and Pricing 
Test Method
Exome Sequencing with CNV DetectionTest Code | Test Copy Genes | Panel CPT Code | Gene CPT Codes Copy CPT Code | Base Price | |
---|---|---|---|---|---|
10191 | Genes x (7)![]() | 81479 | 81222(x1), 81223(x1), 81404(x2), 81405(x2), 81479(x8) | $1090 | Order Options and Pricing |
Pricing Comments
We are happy to accommodate requests for testing single genes in this panel or a subset of these genes. The price will remain the list price. If desired, free reflex testing to remaining genes on panel is available. Alternatively, a single gene or subset of genes can also be ordered via our Custom Panel tool.
An additional 25% charge will be applied to STAT orders. STAT orders are prioritized throughout the testing process.
Click here for costs to reflex to whole PGxome (if original test is on PGxome Sequencing platform).
Click here for costs to reflex to whole PGnome (if original test is on PGnome Sequencing platform).
Turnaround Time
3 weeks on average for standard orders or 2 weeks on average for STAT orders.
Please note: Once the testing process begins, an Estimated Report Date (ERD) range will be displayed in the portal. This is the most accurate prediction of when your report will be complete and may differ from the average TAT published on our website. About 85% of our tests will be reported within or before the ERD range. We will notify you of significant delays or holds which will impact the ERD. Learn more about turnaround times here.
Targeted Testing
For ordering sequencing of targeted known variants, go to our Targeted Variants page.
Clinical Features and Genetics 
Clinical Features
Pancreatitis is characterized by recurrent episodes of inflammation of the pancreas in both adults and children (Chen and Ferec 2009). Symptoms usually begin in late childhood with an episode of acute pancreatitis and include severe upper abdominal burning pain radiating to the back, nausea, and vomiting that is worsened with eating (acute pancreatitis). Recurrent acute pancreatitis leads to chronic pancreatitis due to persistent inflammation. Chronic pancreatitis usually develops by early adulthood in affected individuals and symptoms include occasional or frequent abdominal pain of varying severity, flatulence, and bloating. Unexplained weight loss may occur from a lack of pancreatic enzymes hindering digestion (Rebours et al. 2012). Episodes of pancreatitis can lead to permanent tissue damage and loss of pancreatic function. Chronic pancreatitis increases the risk for diabetes and pancreatic cancer, more so with smoking and use of alcohol (Yadav and Whitcomb 2010). Mutations in several genes have been identified to be causative for, or induce susceptibility to pancreatitis (Joergensen et al. 2010). Genetic testing can aid in differential diagnosis of chronic pancreatitis from other disorders such as Shwachman-Diamond syndrome and Johanson-Blizzard syndrome which also present with pancreatitis (LaRusch et al. 2014).
Genetics
Hereditary chronic pancreatitis (HP) occurs at an estimated incidence of 0.3/100,000 in western countries (Joergensen et al. 2010) and is inherited in an autosomal dominant manner through mutations in the PRSS1 gene in ~60% of cases. About 30% of HP results from inheritance with reduced penetrance through mutations in the SPINK1, CFTR, CTRC, and CASR genes (Masson et al. 2013; LaRusch et al. 2014).
PRSS1 encodes trypsinogen which becomes converted to active trypsin after secretion into the duodenum to aid in digestion. About 60% of HP cases are inherited in an autosomal dominant manner through mutations in the PRSS1 gene. Pathogenic mutations typically result in premature conversion of trypsinogen or resistance to degradation. Duplication in the PRSS1 gene resulting in elevated trypsinogen levels are also a reported cause to HP (Chen and Ferec et al. 2009; LaRusch et al. 2014).
SPINK1 encodes a pancreatic secretory trypsin inhibitor which protects the pancreas from damage due to recurrent or persistent trypsin activation (Horii et al. 1987). HP can be inherited in an autosomal recessive manner through SPINK1 mutations or more commonly through a possible digenic mode together with mutations in the CFTR and CTRC genes. Splice site alterations, gross deletions, and frameshift mutations are considered severe alleles with the highest penetrance in the SPINK1 gene. However, a moderate penetrant missense mutation, c.101A>G (p.Asn34Ser), is most commonly found within SPINK1 in European and North American populations. The c.194+2T>C variant is commonly found in Asian populations (LaRusch et al. 2014).
CFTR encodes a transmembrane conductance protein which functions to secrete bicarbonate fluid to flush pancreatic zymogens into the duodenum. HP can be inherited through an autosomal recessive manner through CFTR mutations but more commonly through a more complex mode together with mutations in the SPINK1 or CASR genes. CFTR mutations have been reported in the presence of PRSS1 mutations in a minor subset of HP patients (Masson et al. 2013).
CTRC encodes chymotrypsin C which degrades prematurely activated trypsinogen within the pancreas. To date, CTRC mutations have primarily been reported in HP patients with co-occurring CFTR mutations, but may co-occur with SPINK1 mutations. Loss of function mutations are primarily found in the CTRC gene in patients with HP (Masson et al. 2013).
CASR encodes a calcium sensing receptor that maintains calcium homeostasis. CASR mutation are low penetrant and are only found in HP patients together with CFTR or SPINK1 mutations (Whitcomb et al. 2013 Felderbauer et al. 2006).
For further information on PRSS1, SPINK1, CFTR, CTRC, or CASR genes please refer to the individual test descriptions.
Clinical Sensitivity - Sequencing with CNV PGxome
About 48% of idiopathic chronic pancreatitis patients were found to display evidence of a genetic basis for their pancreatitis and carried at least one abnormal allele in one of the four genes, PRSS1, SPINK1, CFTR or CTRC (Masson et al. 2013).
Though rare, complete gene duplications have been reported for PRSS1-associated HP (Masson et al. 2008; Le Maréchal et al. 2006). To date, no gross duplications or deletions have been reported for CFTR-associated pancreatitis (Human Gene Mutation Database).
Testing Strategy
This test is performed using Next-Gen sequencing with additional Sanger sequencing as necessary.
This panel provides 100% coverage of all coding exons of the genes plus 10 bases of flanking noncoding DNA in all available transcripts along with other non-coding regions in which pathogenic variants have been identified at PreventionGenetics or reported elsewhere. This test also includes sequencing of CFTR regions within intron 11 and intron 21, along with a complete analysis of the compound (TG)n(T)n sequence (5T/TG tract) in intron 8. Variations in the 5T/TG tract have been reported to cause CBAVD (Chillon et al. 1995). Our 5T/TG tract analysis involves bidirectional sequencing along with allele length measurement. We define coverage as ≥20X NGS reads or Sanger sequencing. PGnome panels typically provide slightly increased coverage over the PGxome equivalent. PGnome sequencing panels have the added benefit of additional analysis and reporting of deep intronic regions (where applicable).
Dependent on the sequencing backbone selected for this testing, discounted reflex testing to any other similar backbone-based test is available (i.e., PGxome panel to whole PGxome; PGnome panel to whole PGnome).
Indications for Test
Individuals with the following criteria should consider genetic testing (Ellis et al. 2001): recurrent unexplained attacks of acute pancreatitis and a positive family history, unexplained chronic pancreatitis and a positive family history, unexplained chronic pancreatitis without a positive family history after exclusion of other causes such as hyperlipidaemia type I, familiar hypercalciuric hypercalcemia (FBH), hereditary hyperthyroidism and autoimmune pancreatitis, and unexplained pancreatitis episodes in children.
Individuals with the following criteria should consider genetic testing (Ellis et al. 2001): recurrent unexplained attacks of acute pancreatitis and a positive family history, unexplained chronic pancreatitis and a positive family history, unexplained chronic pancreatitis without a positive family history after exclusion of other causes such as hyperlipidaemia type I, familiar hypercalciuric hypercalcemia (FBH), hereditary hyperthyroidism and autoimmune pancreatitis, and unexplained pancreatitis episodes in children.
Genes
Official Gene Symbol | OMIM ID |
---|---|
CASR | 601199 |
CFTR | 602421 |
CPA1 | 114850 |
CTRC | 601405 |
PRSS1 | 276000 |
SPINK1 | 167790 |
TRPV6 | 606680 |
Inheritance | Abbreviation |
---|---|
Autosomal Dominant | AD |
Autosomal Recessive | AR |
X-Linked | XL |
Mitochondrial | MT |
Diseases
Name | Inheritance | OMIM ID |
---|---|---|
Hyperparathyroidism, transient neonatal | AR | 618188 |
Pancreatitis, Chronic | AD | 167800 |
Related Test
Name |
---|
PGxome® |
Citations 
- Chen J-M, Férec C. 2009. Chronic pancreatitis: genetics and pathogenesis. Annu Rev Genomics Hum Genet 10: 63–87. PubMed ID: 19453252
- Chillon, M, Casals, T, Mercier, B, Bassas, L, Lissens, W, Silber, S, Romey, MC, Ruiz-Romero, J, Verlingue, C, Claustres, M, et al. 1995. Mutations in the cystic fibrosis gene in patients with congenital absence of the vas deferens. N. Engl. J. Med. 332: 1475-1480. PubMed ID: 7739684
- Ellis, I, Lerch, MM, Whitcomb, DC. 2001. Genetic testing for hereditary pancreatitis: guidelines for indications, counselling, consent and privacy issues. Pancreatology 1:405-415. PubMed ID: 12120217
- Felderbauer P, Klein W, Bulut K, Ansorge N, Dekomien G, Werner I, Epplen JT, Schmitz F, Schmidt WE. 2006. Mutations in the calcium-sensing receptor: a new genetic risk factor for chronic pancreatitis? Scand. J. Gastroenterol. 41: 343–348. PubMed ID: 16497624
- Horii A, Kobayashi T, Tomita N, Yamamoto T, Fukushige S, Murotsu T, Ogawa M, Mori T, Matsubara K. 1987. Primary structure of human pancreatic secretory trypsin inhibitor (PSTI) gene. Biochem. Biophys. Res. Commun. 149: 635–641. PubMed ID: 3501289
- Human Gene Mutation Database (Bio-base).
- Joergensen, MT, Brusgaard, K, Crüger, DG, Gerdes, AM, Schaffalitzky de Muckadell, OB. 2010. Genetic, epidemiological, and clinical aspects of hereditary pancreatitis: a population-based cohort study in Denmark. Am. J. Gastroenterol. 105:1876-1883. PubMed ID: 20502448
- LaRusch J, Solomon S, Whitcomb DC. 2014. Pancreatitis Overview. In: Pagon RA, Adam MP, Ardinger HH, Bird TD, Dolan CR, Fong C-T, Smith RJ, and Stephens K, editors. GeneReviews(®), Seattle (WA): University of Washington, Seattle. PubMed ID: 24624459
- Le Maréchal C, Masson E, Chen J-M, Morel F, Ruszniewski P, Levy P, Férec C. 2006. Hereditary pancreatitis caused by triplication of the trypsinogen locus. Nat. Genet. 38: 1372–1374. PubMed ID: 17072318
- Masson E, Maréchal C Le, Chandak GR, Lamoril J, Bezieau S, Mahurkar S, Bhaskar S, Reddy DN, Chen J-M, Férec C. 2008. Trypsinogen copy number mutations in patients with idiopathic chronic pancreatitis. Clin. Gastroenterol. Hepatol. 6: 82–88. PubMed ID: 18063422
- Masson, E, Chen, JM, Audrézet, MP, Cooper, DN, Férec, C. 2013. A Conservative Assessment of the Major Genetic Causes of Idiopathic Chronic Pancreatitis: Data from a Comprehensive Analysis of PRSS1, SPINK1, CTRC and CFTR Genes in 253 Young French Patients. PLoS One 8:e73522. PubMed ID: 23951356
- Rebours V, Lévy P, Ruszniewski P. 2012. An overview of hereditary pancreatitis. Dig Liver Dis 44: 8–15. PubMed ID: 21907651
- Whitcomb DC. 2013. Genetic Risk Factors for Pancreatic Disorders. Gastroenterology 144: 1292–1302. PubMed ID: 23622139
- Yadav D, Whitcomb DC. 2010. The role of alcohol and smoking in pancreatitis. Nat Rev Gastroenterol Hepatol 7: 131–145. PubMed ID: 20125091
Ordering/Specimens 
Ordering Options
We offer several options when ordering sequencing tests. For more information on these options, see our Ordering Instructions page. To view available options, click on the Order Options button within the test description.
myPrevent - Online Ordering
- The test can be added to your online orders in the Summary and Pricing section.
- Once the test has been added log in to myPrevent to fill out an online requisition form.
- PGnome sequencing panels can be ordered via the myPrevent portal only at this time.
Requisition Form
- A completed requisition form must accompany all specimens.
- Billing information along with specimen and shipping instructions are within the requisition form.
- All testing must be ordered by a qualified healthcare provider.
For Requisition Forms, visit our Forms page
If ordering a Duo or Trio test, the proband and all comparator samples are required to initiate testing. If we do not receive all required samples for the test ordered within 21 days, we will convert the order to the most effective testing strategy with the samples available. Prior authorization and/or billing in place may be impacted by a change in test code.
Specimen Types
Specimen Requirements and Shipping Details
PGxome (Exome) Sequencing Panel
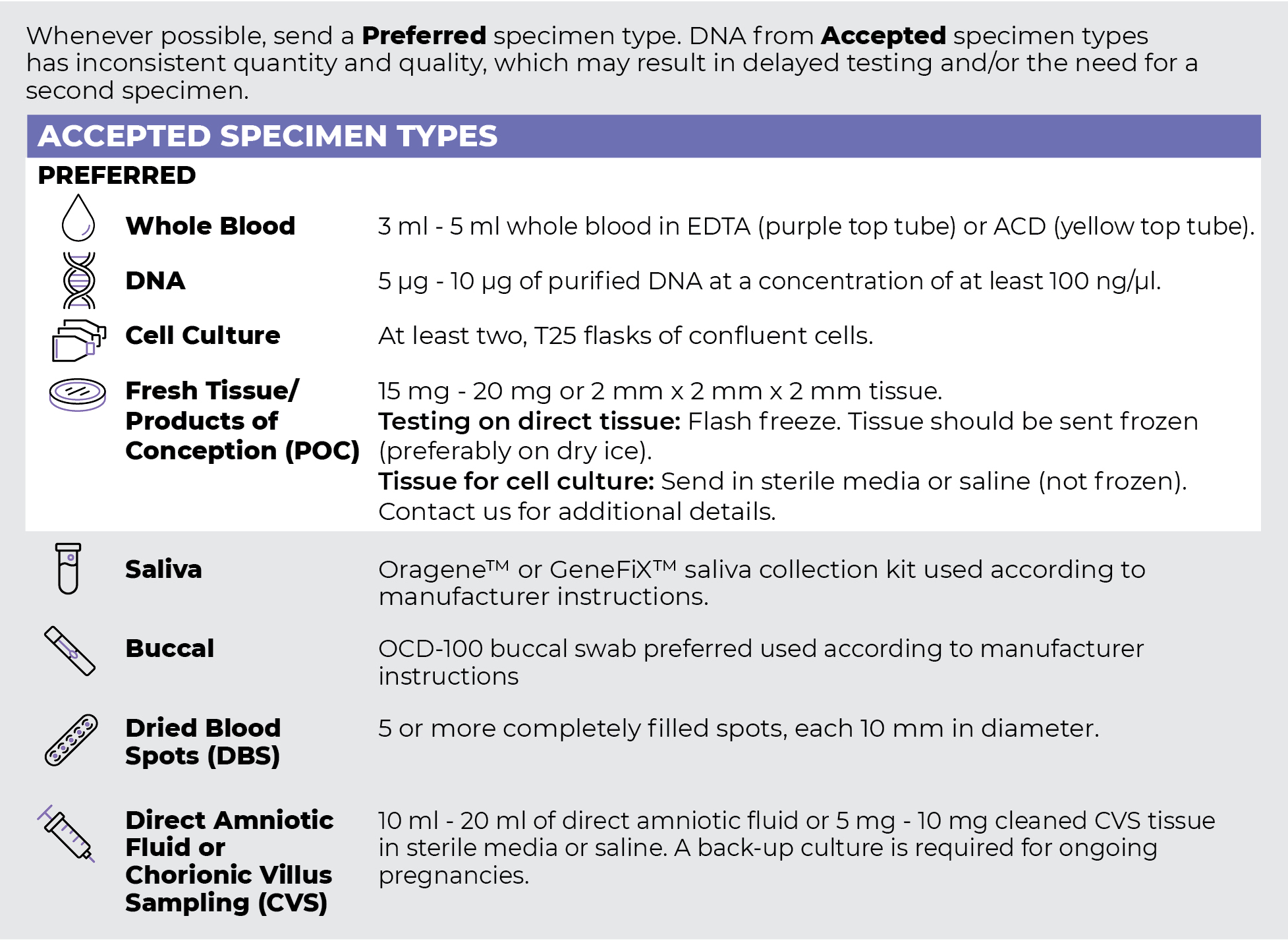
PGnome (Genome) Sequencing Panel
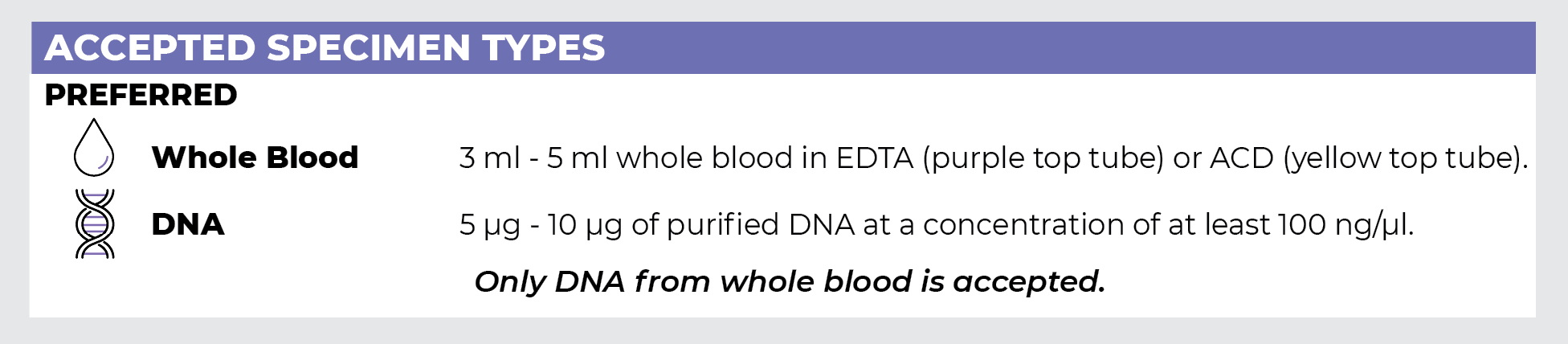
ORDER OPTIONS
View Ordering Instructions1) Select Test Type
2) Select Additional Test Options
No Additional Test Options are available for this test.