Antithrombin Deficiency via the SERPINC1 Gene
Summary and Pricing 
Test Method
Exome Sequencing with CNV DetectionTest Code | Test Copy Genes | Test CPT Code | Gene CPT Codes Copy CPT Code | Base Price | |
---|---|---|---|---|---|
8369 | SERPINC1 | 81479 | 81479,81479 | $990 | Order Options and Pricing |
Pricing Comments
Our favored testing approach is exome based NextGen sequencing with CNV analysis. This will allow cost effective reflexing to PGxome or other exome based tests. However, if full gene Sanger sequencing is desired for STAT turnaround time, insurance, or other reasons, please see link below for Test Code, pricing, and turnaround time information. If the Sanger option is selected, CNV detection may be ordered through Test #600.
An additional 25% charge will be applied to STAT orders. STAT orders are prioritized throughout the testing process.
Click here for costs to reflex to whole PGxome (if original test is on PGxome Sequencing platform).
Click here for costs to reflex to whole PGnome (if original test is on PGnome Sequencing platform).
The Sanger Sequencing method for this test is NY State approved.
For Sanger Sequencing click here.Turnaround Time
3 weeks on average for standard orders or 2 weeks on average for STAT orders.
Please note: Once the testing process begins, an Estimated Report Date (ERD) range will be displayed in the portal. This is the most accurate prediction of when your report will be complete and may differ from the average TAT published on our website. About 85% of our tests will be reported within or before the ERD range. We will notify you of significant delays or holds which will impact the ERD. Learn more about turnaround times here.
Targeted Testing
For ordering sequencing of targeted known variants, go to our Targeted Variants page.
Clinical Features and Genetics 
Clinical Features
Antithrombin deficiency (AD) is a disorder of elevated blood clotting. An estimated 1 in 600 to 1 in 5000 individuals have AD (Harper et al. 1991; Tait et al. 1994). While many patients are asymptomatic until later in life, individuals with AD are at heightened risks for venous thromboembolism (VTE). VTE is a major cause of morbidity and death in hospitalized patients (Heit et al. 2001) and maternal death in pregnancy (James et al. 2013). About half of people with AD will develop at least one abnormal blood clot during their lifetime, often after adolescence. In severe cases clotting may occur during childhood. Environmental factors including surgery, increased age, and immobility heighten risk for VTE.
Antithrombin protein regulates blood clotting by inactivating factors that facilitate clotting such as thrombin. There are two types of AD. Type I AD deficiency is characterized by loss of both AD protein activity and levels within the blood. Type II, the more common form, presents with normal protein levels, but impaired activity. AD concentrates, low molecular weight heparin, and other anticoagulant therapies have been used to treat disease. Diagnosis can be difficult as commercial kits assaying antithrombin activity vary widely (Javela et al. 2013), and secondary pathologies such as sepsis result in acquired AD (White and Perry 2001).
Genetics
Antithrombin deficiency is inherited in an autosomal dominant manner with variable penetrance cause by mutations in the SERPINC1 gene. Risk of VTE development is highly correlated to degree of antithrombin loss of function. Type I deficiency is primarily caused through nonsense or small insertion/deletion mutations resulting in frameshift and premature protein termination (Cooper et al. 2011). Missense mutations altering glycosylation and leading to intracellular retention of antithrombin have also been described in individuals with type I deficiency (Fitches et al. 2001; Beauchamp et al. 1998). Type II deficiency is primarily caused by missense mutations disrupting enzyme-inhibitor and heparin binding sites, although mutations are found throughout the coding region. Large deletions are found in <10% of individuals with type I deficiency. Patients homozygous and compound heterozygous for AD defects have been reported in a small number of cases. Such mutations are associated with severe thromboembolic disease (Kuhle et al. 2001; Picard et al. 2010). Complete deficiency in antithrombin is incompatible with life (Ishiguro et al. 2000). Antithrombin is a serine protease inhibitor found freely in blood plasma. It inactivates pro-coagulation proteins, primarily thrombin and factor X, to prevent excessive clot formation and is a primary factor for dissolution of clots after vessel repair.
Clinical Sensitivity - Sequencing with CNV PGxome
Clinical sensitivity should be high as two independent reports identified causative SERPINC1 mutations in 23 of 23 and 18 of 18 patients with a prior diagnosis with hereditary AD (van Boven et al. 1994; Picard et al. 2006). In a cohort of 150 patients with a prior VTE history, ~4% of individuals had mutations in the SERPINC1 gene (Fischer et al. 2013). Analytical sensitivity is ~90% as large deletions in the SERPINC1 gene have been reported in a minor subset of AD patients.
Testing Strategy
This test provides full coverage of all coding exons of the SERPINC1 gene plus 10 bases of flanking noncoding DNA in all available transcripts along with other non-coding regions in which pathogenic variants have been identified at PreventionGenetics or reported elsewhere. We define full coverage as >20X NGS reads or Sanger sequencing. PGnome panels typically provide slightly increased coverage over the PGxome equivalent. PGnome sequencing panels have the added benefit of additional analysis and reporting of deep intronic regions (where applicable).
Dependent on the sequencing backbone selected for this testing, discounted reflex testing to any other similar backbone-based test is available (i.e., PGxome panel to whole PGxome; PGnome panel to whole PGnome).
Indications for Test
Patients with family history of VTE and pregnancy loss are candidates for testing. Ideal candidates have diagnostic data indicating impaired antithrombin function or levels. Genetic testing is a strong tool to distinguish between acquired and inherited forms of AD (Cooper et al. 2011; Patnaik and Moll 2008).
Patients with family history of VTE and pregnancy loss are candidates for testing. Ideal candidates have diagnostic data indicating impaired antithrombin function or levels. Genetic testing is a strong tool to distinguish between acquired and inherited forms of AD (Cooper et al. 2011; Patnaik and Moll 2008).
Gene
Official Gene Symbol | OMIM ID |
---|---|
SERPINC1 | 107300 |
Inheritance | Abbreviation |
---|---|
Autosomal Dominant | AD |
Autosomal Recessive | AR |
X-Linked | XL |
Mitochondrial | MT |
Disease
Name | Inheritance | OMIM ID |
---|---|---|
Antithrombin III Deficiency | AR, AD | 613118 |
Citations 
- Beauchamp NJ, Pike RN, Daly M, Butler L, Makris M, Dafforn TR, Zhou A, Fitton HL, Preston FE, Peake IR, Carrell RW. 1998. Antithrombins Wibble and Wobble (T85M/K): archetypal conformational diseases with in vivo latent-transition, thrombosis, and heparin activation. Blood 92: 2696–2706. PubMed ID: 9763552
- Cooper PC, Coath F, Daly ME, Makris M. 2011. The phenotypic and genetic assessment of antithrombin deficiency. Int J Lab Hematol 33: 227–237. PubMed ID: 21401902
- Fischer R, Sachs UJ, Heidinger KS, Eisenburger D, Kemkes-Matthes B. 2013. Prevalence of hereditary antithrombin mutations is higher than estimated in patients with thrombotic events. Blood Coagul. Fibrinolysis 24: 444–448. PubMed ID: 23429250
- Fitches AC, Lewandowski K, Olds RJ. 2001. Creation of an additional glycosylation site as a mechanism for type I antithrombin deficiency. Thromb. Haemost. 86: 1023–1027. PubMed ID: 11686319
- Harper PL, Luddington RJ, Daly M, Bruce D, Williamson D, Edgar PF, Perry DJ, Carrell RW. 1991. The incidence of dysfunctional antithrombin variants: four cases in 210 patients with thromboembolic disease. Br. J. Haematol. 77: 360–364. PubMed ID: 2012760
- Heit JA, Melton LJ 3rd, Lohse CM, Petterson TM, Silverstein MD, Mohr DN, O’Fallon WM. 2001. Incidence of venous thromboembolism in hospitalized patients vs community residents. Mayo Clin. Proc. 76: 1102–1110. PubMed ID: 11702898
- Ishiguro K, Kojima T, Kadomatsu K, Nakayama Y, Takagi A, Suzuki M, Takeda N, Ito M, Yamamoto K, Matsushita T, Kusugami K, Muramatsu T, et al. 2000. Complete antithrombin deficiency in mice results in embryonic lethality. J. Clin. Invest. 106: 873–878. PubMed ID: 11018075
- James AH, Konkle BA, Bauer KA. 2013. Prevention and treatment of venous thromboembolism in pregnancy in patients with hereditary antithrombin deficiency. Int J Womens Health 5: 233–241. PubMed ID: 23662090
- Javela K, Engelbarth S, Hiltunen L, Mustonen P, Puurunen M. 2013. Great discrepancy in antithrombin activity measured using five commercially available functional assays. Thromb. Res. 132: 132–137. PubMed ID: 23768451
- Kuhle S, Lane DA, Jochmanns K, Male C, Quehenberger P, Lechner K, Pabinger I. 2001. Homozygous antithrombin deficiency type II (99 Leu to Phe mutation) and childhood thromboembolism. Thromb. Haemost. 86: 1007–1011. PubMed ID: 11686316
- Patnaik MM, Moll S. 2008. Inherited antithrombin deficiency: a review. Haemophilia 14: 1229–1239. PubMed ID: 19141163
- Picard V, Chen J-M, Tardy B, Aillaud M-F, Boiteux-Vergnes C, Dreyfus M, Emmerich J, Lavenu-Bombled C, Nowak-Göttl U, Trillot N, Aiach M, Alhenc-Gelas M. 2010. Detection and characterisation of large SERPINC1 deletions in type I inherited antithrombin deficiency. Hum. Genet. 127: 45–53. PubMed ID: 19760264
- Picard V, Nowak-Göttl U, Biron-Andreani C, Fouassier M, Frere C, Goualt-Heilman M, Maistre E de, Regina S, Rugeri L, Ternisien C, Trichet C, Vergnes C, et al. 2006. Molecular bases of antithrombin deficiency: twenty-two novel mutations in the antithrombin gene. Hum. Mutat. 27: 600. PubMed ID: 16705712
- Tait RC, Walker ID, Perry DJ, Islam SI, Daly ME, McCall F, Conkie JA, Carrell RW. 1994. Prevalence of antithrombin deficiency in the healthy population. Br. J. Haematol. 87: 106–112. PubMed ID: 7947234
- van Boven HH, Olds RJ, Thein SL, Reitsma PH, Lane DA, Briët E, Vandenbroucke JP, Rosendaal FR. 1994. Hereditary antithrombin deficiency: heterogeneity of the molecular basis and mortality in Dutch families. Blood 84: 4209–4213. PubMed ID: 7994035
- White B, Perry D. 2001. Acquired antithrombin deficiency in sepsis. Br. J. Haematol. 112: 26–31. PubMed ID: 11167778
Ordering/Specimens 
Ordering Options
We offer several options when ordering sequencing tests. For more information on these options, see our Ordering Instructions page. To view available options, click on the Order Options button within the test description.
myPrevent - Online Ordering
- The test can be added to your online orders in the Summary and Pricing section.
- Once the test has been added log in to myPrevent to fill out an online requisition form.
- PGnome sequencing panels can be ordered via the myPrevent portal only at this time.
Requisition Form
- A completed requisition form must accompany all specimens.
- Billing information along with specimen and shipping instructions are within the requisition form.
- All testing must be ordered by a qualified healthcare provider.
For Requisition Forms, visit our Forms page
If ordering a Duo or Trio test, the proband and all comparator samples are required to initiate testing. If we do not receive all required samples for the test ordered within 21 days, we will convert the order to the most effective testing strategy with the samples available. Prior authorization and/or billing in place may be impacted by a change in test code.
Specimen Types
Specimen Requirements and Shipping Details
PGxome (Exome) Sequencing Panel
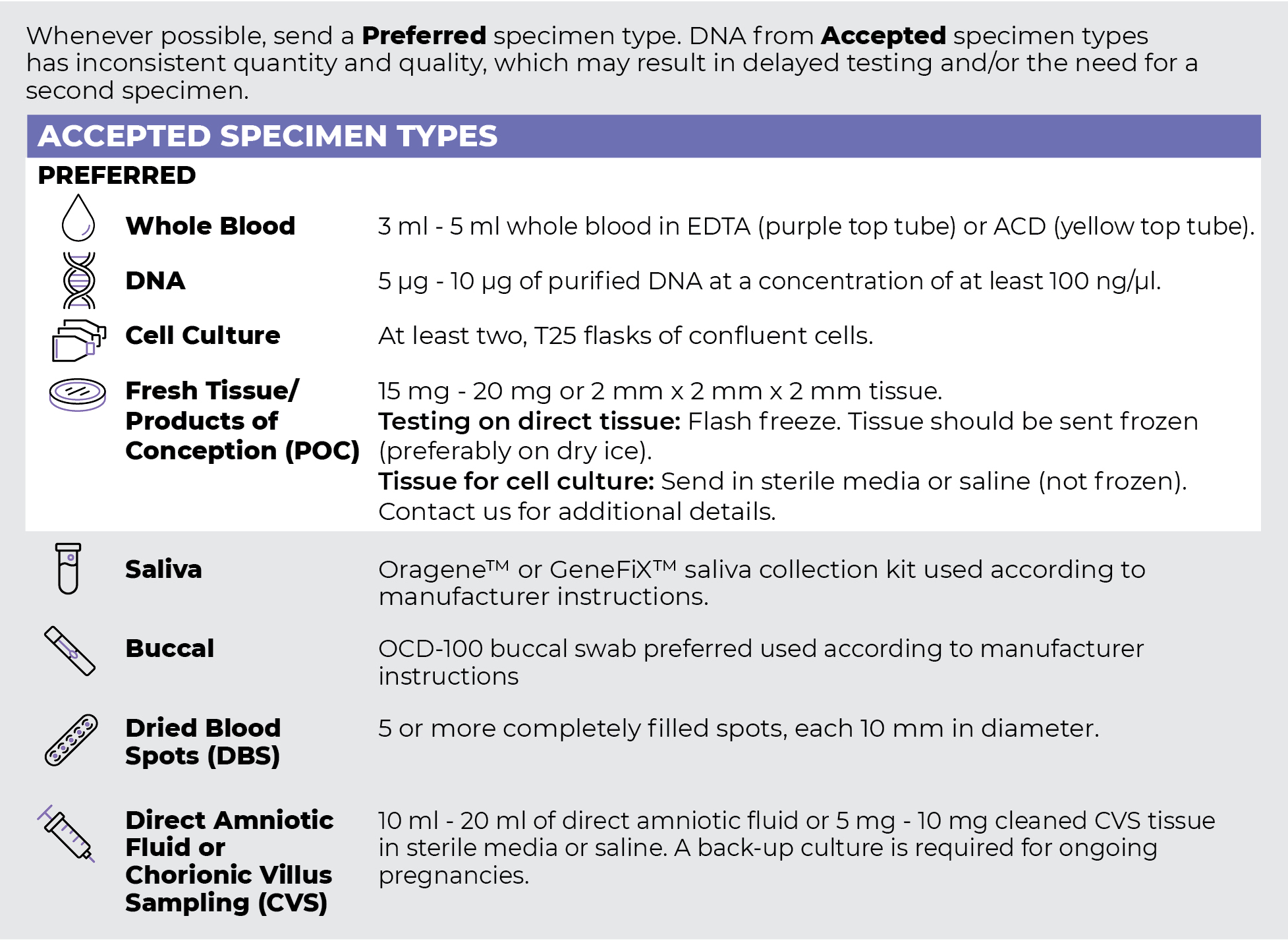
PGnome (Genome) Sequencing Panel
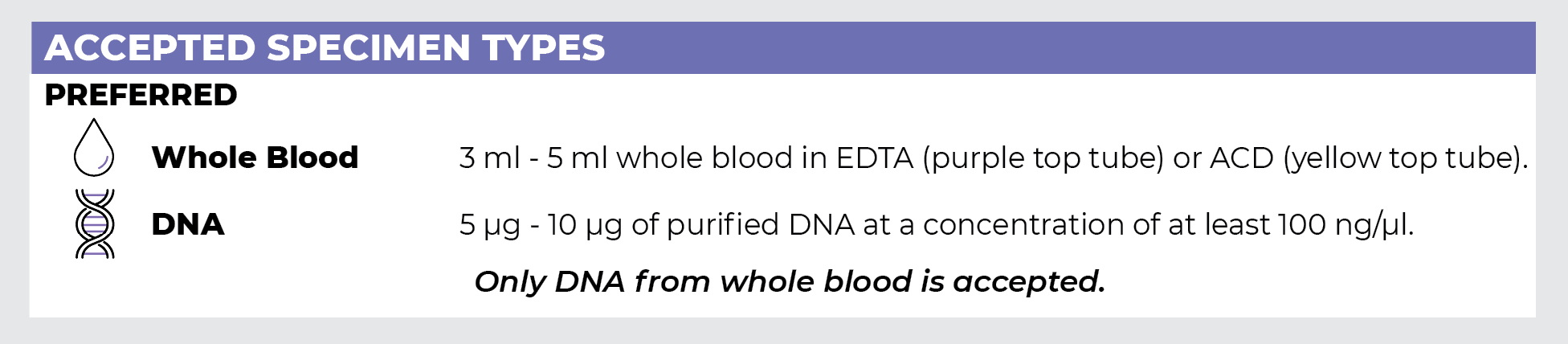
ORDER OPTIONS
View Ordering Instructions1) Select Test Type
2) Select Additional Test Options
No Additional Test Options are available for this test.